Reaching new heights with high-throughput flow cytometry
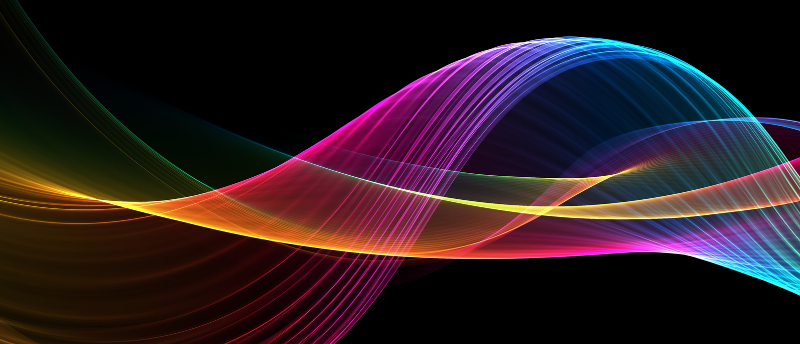
Larry Sklar (left) is a Distinguished Professor Emeritus at the University of New Mexico (UNM; NM, USA) whose interest in flow cytometry as a tool for drug discovery led to the development of high-throughput flow cytometry technologies and their applications to drug discovery for a number of diseases. In this interview for our Spotlight on antibody discovery, we talk to Larry about how he developed the technologies, how they can be utilized in drug discovery and how the field has evolved since then.
Could you please provide a brief summary of your career to date?
My degrees include a bachelor’s degree in chemistry and a PhD in physical chemistry. That work, as well as two postdoc positions, used fluorescence to study the structure and organization of biological membranes, lipoproteins and lipid–protein interactions. For my first faculty position, I joined an immunology group in a department at Scripps Research (CA, USA) that used early commercial versions of flow cytometers. My mandate was to think about how physical chemistry approaches could be used to acquire data from living cells. One of our projects involved white cells and inflammation, particularly how inflammatory ligands bound to receptors on white cells. I learned that those interactions could be studied with a fluorescent ligand in a fluorometer or a flow cytometer in real-time – as it was occurring. I learned that it was possible to acquire these measurements in a homogeneous way, without washing away the unbound fluorescent ligand from the cells. In the flow cytometer, we could watch the cells get brighter over time as the ligand bound. I was motivated to pursue new applications of flow cytometry and instrumentation improvements that would allow us to use flow cytometry to look at ligand-binding events – the initiating events – and the cellular responses that follow on an appropriate time frame. I accepted joint positions at the University of New Mexico in Albuquerque (UNM; NM, USA) and as director of the National Flow Cytometry Resource at Los Alamos National Laboratory (LANL; NM, USA) to pursue these opportunities.
You invented the high-throughput flow cytometry platform HyperCyt. What was the rationale for developing the platform?
My first project at LANL was to build a stopped-flow or rapid mix flow cytometer, which ultimately involved Rich Posner (Northern Arizona University; AZ, USA), John Nolan (Scintillon Institute; CA, USA) and Steve Graves (UNM). The instrument could deliver samples to be analyzed in a sub-second time frame. John Nolan and I described the role that flow cytometry was already playing in many phases of drug discovery. I began teaching a series of lectures to grad students at UNM about G protein-coupled receptors and drug discovery. For the lectures, I collected a number of publications about screening and thought about how it could be done using flow cytometry. We had to convert a fast measurement into sequential measurements that could be repeated rapidly using small volumes. An appropriate starting point was to think about sampling from other systems such as viscometers and multi-well plates as Bruce Edwards, Fritz Kuckuck and I were learning to do at UNM. We tried a number of approaches whose prototypes could literally fill a junkyard. We understood that the homogeneous discrimination of free and bound ligand and the potential for using multi-parametric analysis would create significant advantages: no wash sample delivery; analysis of multiple targets simultaneously on cell and microspheres; analysis of cell subsets as targets; as well as the ability to interrogate pathogen responses and host-pathogen interactions for applications in infectious diseases.
How does the platform work?
The platform connects an autosampler to a flow cytometer using a peristaltic pump to deliver the sample from multi-well plates. The autosampler ‘sips’ (picks up) a sample from each well (~1microliter) and inserts an air bubble as the sample moves from well to well. The entire plate is delivered as a single sample, with the software identifying the samples from individual wells. The analysis of the data from the individual wells can be automated and batched for large data sets in high-throughput screen. The sampling can be enhanced through shaking the plate to keep the samples in individual wells suspended, by having the temperature controlled, by programming the movement of the autosampler tip to allow for changing the sip time (to adjust the number of cells or particles), as well as by changing the frequency of blank or control samples (typically at the ends of each row or column) on the plate.
High-throughput flow cytometry is used in institutions and companies around the world. What do you think has led to the technology being so successful?
During the time that the technology was introduced by the local startup company IntelliCyt (NM, USA) and prior to acquisition by Sartorius (Göttingen, Germany), the inventors at UNM continued to develop applications for early adopters. We were participants in the National Institutes of Health Molecular Libraries Program so the technology got significant exposure along the way and we also received infrastructure support in what became known as the UNM Center for Molecular Discovery. There was a pent-up demand in the research community for taking advantage of flow cytometry with higher throughput, which included bead-based assays, antibody assays and cell-based assays. The community understand the advantages that were complimentary to existing technologies and include analysis of samples in suspension with high rate of sample analysis (thousands of events per second), no wash discrimination of free and bound molecules (ligand, antibody, etc), multiparameter analysis translating to multiplexed target screening, cell subsets and cell–cell interactions. This is to say that essentially any assay that could be done by flow cytometry had the potential to be performed in high throughput. Early expertise in data analysis and data display both at UNM and the startup was led by co-inventor Bruce Edwards, an immunologist with instrumentation and programming expertise.
High-throughput flow cytometry has a wide range of applications. How is it used in drug discovery and development?
The technology can be used in primary screening and high-throughput screening for novel molecules, repurposed molecules, antibody discovery, precision medicine and immuno-oncology using patient samples, as well as in infectious diseases. Validation of compounds can readily be performed in secondary or dose-response screening, as well as orthogonal assays for off-target effects and analysis of fluorescence artifacts. The technology is also compatible with whole blood analysis for the action of compounds on white blood cell; detection of cytokines and other analytes; and detection of rare cell events, such as minimal residual disease in blood cancers.
Representative additional applications have been described the book Flow Cytometry in Drug Discovery and Development [1] as well as a special volume of SLAS Discovery [2]. These include cell cycle analysis in oncology; preclinical toxicology and safety; drug target inhibition in laboratory animals and early phase clinical trials; CD4 T-cell analysis in HIV therapeutics; monitoring the responses of immune cells during clinical trials; immunogenicity testing, pharmacokinetics; regulatory compliance and method validation. Pathway analysis is also amenable through phosphoproteins detected with antibodies (‘phos-flow’).
How has the drug discovery field evolved since it was developed?
Drug discovery has a history that involves animal, organ-based, cell and molecular targets, as well as phenotypic assays. Flow cytometry can contribute to both cell and molecular targets as well as cell phenotype assays. The assays can be made more robust when several response parameters can be analyzed in a ratio mode. Over the last few decades, drug discovery has evolved from screening large compound libraries, to screening targeted libraries. In principal, the targeted libraries and iterative processes can take advantage of an interplay between chemical, computational, biological and structural tools. Some envision that these processes can be automated at multiple stages with the application of machine learning and artificial intelligence.
How do you see the field developing in the future?
I see applications both to precision medicine, based on the behavior of patient samples treated with targeted libraries, as well as infectious diseases, where working with pathogens in suspension will be useful. In fact, we have reported the integration of high-throughput flow cytometry into biosafety level-3 facilities. The multiparameter aspects of flow cytometry will expand for the analysis of multiple targets as well as responding cell subsets. In the case of multiple targets, primary screens will provide information about selectivity of the molecules screened against targets and phenotypes. Likewise, in the case of cell subsets, it will be possible to identify, quantify and target responding populations.
Is there anything else you’d like to add?
Over several decades, contributions to the development and applications of the platform have involved dozens of people at UNM, and hundreds if not thousands worldwide. There are several additional features of flow cytometry that are advantageous for drug discovery. First, the assessment of binding interactions is facilitated by the presence of the binding partner on a cell or microsphere surface. If the surface density of the partner is 10,000 per particle and the particle concentration is 1000 per microliter, the concentration of the binding partner is about 10 picomolar. Typically, binding interactions in solution need to be analyzed near the dissociation constant (Kd) of the interaction, which can range as high as micromolar concentrations. In the flow cytometer, the reaction is driven by the free fluorescent ligand, whereas in suspension each reagent must typically be present near the Kd. The display of the expensive partner on the particle has the potential of reducing reagent costs for screening by orders of magnitude. Families of binding interactions can be studied in a multiplexed manner.
Secondly, the homogeneous discrimination of free and bound ligand is useful within concentration ranges that depend on the autofluorescence of the cell or particle, as well as the number of binding sites, the Kd and non-specific binding. However, as a rule of thumb, homogeneous binding has the potential for being detected at 1000 sites per particles when the Kd is 1 nanomolar and ranging to 1,000,000 sites when the Kd is 1 micromolar. Third, anecdotally, we have observed that GFP-based assays for protein expression in cells may be more robust in terms of signal to noise by flow cytometry than comparable plate-based adherent cell assays. Assay robustness may also be improved when cytometric parameters can be ratioed.
Finally, in the recent BioTechniques webinar about antibody discovery and antibody-based analysis, a question was asked about comparing the muti-well iQue platform to surface plasmon resonance where binding kinetics can be followed. It is worthwhile noting that the sample analysis in flow cytometry is eminently compatible with kinetics of fluorescent ligand or antibody binding and dissociation. In principle, a multi-well approach to antibody kinetics could be achieved with repetitive, timed sampling of individual wells across a plate.