Can learning outside the classroom improve education in synthetic biology?
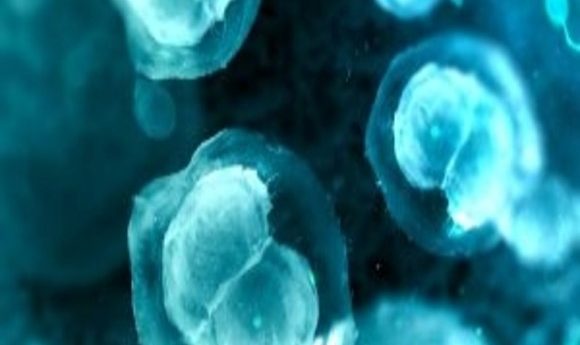
Bill Jia, Arin Wongprommoon and Andre Zylstra from the Cambridge University Synthetic Biology Society (Cambridge, UK) provide a student’s perspective on the importance of being practical and creative for innovation and progress in synthetic biology.
Synthetic biology is a field that aims to develop and construct living systems to perform novel and useful tasks. It exploits techniques such as genetic recombination and gene editing to construct biochemical pathways out of new combinations of synthetic and natural enzymes. One of synthetic biology’s most sought-after goals is to have systems reliable enough to work in the real world.
To achieve this, the discipline often draws inspiration from the practices and techniques of the physical sciences and engineering. Genetic circuits are thought of as combinations of interchangeable sensors and actuators with information flowing between them. Researchers attempt to rationalize behavior and predict it quantitatively with mathematical models, instead of relying entirely on empirical observations.
This interdisciplinary approach has led to creations that would have been unimaginable only a few decades ago, from bacterial digital displays to total synthesis of antimalarials. It will continue to be important for the aspiring synthetic biologist to be able to approach problems from the different perspectives of biology, mathematics, and engineering. The field is rapidly growing, but education and training needs to keep up with its progress.
Undergraduate education in the biological sciences, at least in Europe, is often organized along traditional disciplines or departments. The courses are mainly heavily focused on theoretical knowledge, through lectures and assigning reading lists, without much emphasis on inquiry-based learning. In lab sessions, students spend many contact hours following recipes known to work without being offered much of a chance to problem-solve. If there is teaching of computational methods, it is often by faculty in biological departments rather than faculty in computing departments. The teaching would therefore reflect these academics’ use of these methods as black-box tools: students are instructed to copy-and-paste code and make minor modifications without being offered a solid foundation in the basics of computer science. The current state of how biology is taught in undergraduate courses therefore gets in the way of combining different perspectives from different fields of science, and limits the capacity of using both wet-lab and computational methods in a creative manner to solve problems in synthetic biology.
Giving students the opportunity to ask their own questions can be far more engaging and instructive than most practical experience offered at the undergraduate level. Already commonplace in engineering curricula, the idea is essential to the ethos of innovation in the discipline. A budding electrical engineer can easily and inexpensively buy parts such as diodes and transistors, and use them to fashion anything that might strike her or his imagination. When things don’t work, he or she will ask questions and look for explanations. This cycle of problem solving often makes for a much more tangible and lasting learning experience than the abstract instruction offered in lectures. Engineering programs in most universities actively encourage these experiences through the organization of student teams and the provision of project grants.
Synthetic biology offers a similar cycle of design and tangible realization for the life sciences, and has similar power to educate and inspire. The International Genetically Engineered Machine competition (iGEM) (MA, USA) was born out of this idea and since 2003 has offered thousands of students the opportunity to present their creations on the world stage. Teams have engineered bacteria to act as oxygen-carrying red blood cells, and biosensors to detect arsenic contamination in drinking water, among others. Aside from acting as an educational tool, iGEM has provided an avenue for students to make a significant impact on real research through the Registry of Standard Biological Parts, where teams have contributed over 7000 functional genetic sequences since the program’s inception [1].
Other initiatives have followed in iGEM’s footsteps to provide practical and inventive experiences in synthetic biology for students. Unlike iGEM, which requires at least one faculty member as an advisor, the Cambridge University Synthetic Biology Society (CUSBS) is entirely student-led. Established in 2015, the aim of the society has been to provide learning opportunities and encourage curiosity in synthetic biology for a broader audience. In the past year, the society ran a wet lab project aiming to replicate a bacterial photography system that was a landmark in the field. Students would develop genetic circuits and come into the lab several times a term to try to implement them, learning some experimental techniques and gaining first-hand experience of challenges involved in independent research work that would not be apparent from the lab exercises offered by most university life science courses.
To try to bridge the interdisciplinary gap, CUSBS has also incorporated opportunities for hardware and mathematical modelling in its activities. For the past several years, the society has been working on a 3D-printed automated microscope, born out of the 2015 Cambridge iGEM team’s project. In the spring of 2018, CUSBS also ran a workshop on dynamical systems modelling in Python, where 40 students used mathematics and programming to predict how the lac operon would behave in E. coli under different nutritional conditions. Feedback was overwhelmingly positive, highlighting the strong desire of students in the biological sciences to learn more about quantitative methods. These and other initiatives have also drawn significant interest from students in mathematics and physical sciences, creating an environment where ideas can be exchanged amongst people with disparate backgrounds and new interests can be discovered.
An added benefit of having more open and inclusive avenues for education in synthetic biology is the potential for democratization of the biological sciences. Unlike the inexpensive microprocessors and open-source programming languages at the disposal of the more electronically-inclined hacker, the equipment and materials required for molecular biology are specialized and sometimes hazardous. The costs and safety requirements can make public engagement prohibitively difficult.
But the recent emergence of community hubs for biological experimentation has mitigated some of these problems. Such centers provide a wealth of shared lab equipment, and dedicated staff and volunteers work to allow citizens and hobbyists to participate in science safely and knowledgeably. Among these spaces include the London Bio Hackspace (UK), La Paillasse (Paris, France) and Genspace (NY, USA).
CUSBS works in the recently-established Cambridge Biomakespace (UK). The space is run entirely by a team of volunteers who range from doctorate-holding biologists to hardware hobbyists. Members are offered a fully-equipped lab space for their projects. Biomakespace also runs regular open workshops for interested people with different skills and expertise to gain practical experience. The workshops illustrate techniques such as PCR and next-generation sequencing by examining topics like plant heredity and the origins of human taste. This type of interdisciplinary community building and broader public outreach both have a crucial role in the growth of synthetic biology. They will expand the pool of available talent, help correct misconceptions and raise new questions about the safety and role of genetically modified organisms in society.
The rapidly increasing promise of synthetic biology as a tool for good must be sustained by matched growth in the availability and effectiveness of education in the field. The current state of formal education does not capture the creative and inventive spirit of synthetic biology as effectively as it could, and does not integrate the required knowledge from disparate disciplines. External programs such as iGEM, student societies, and community lab spaces have filled the gap. These initiatives allow students to engage with synthetic biology in a meaningful way, gaining practical and quantitative experience in the process. Schools and universities should take note of what has succeeded, and aim to integrate some of these concepts more closely into mainstream curricula.