Gene-length DNA synthesis for scaling engineering biology
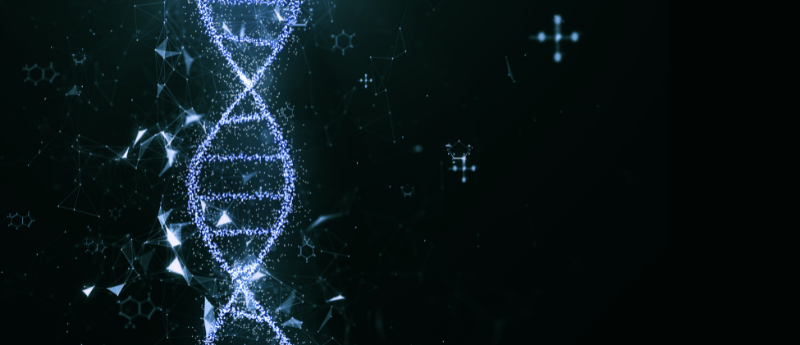
Matthew Hayes (Left) is the Chief Technology Officer and founder at Evonetix, a groundbreaking gene synthesis technology company created in 2015, where he deploys his expertise in multi-disciplinary system design, medical device design, ASIC design and optoelectronics. Before Evonetix, Matthew was Head of Technology for the Global MedTech Division of Cambridge Consultants, with responsibility for the leadership of many of their largest and most challenging projects.
Here, Matthew details the essential role and key challenges of synthetic DNA in engineering biology and reveals Evonetix’s upcoming benchtop platform to deliver the synthesis of gene-length DNA at unprecedented accuracy, scale, and speed. This technology embodies the company’s ambition to free scientists from the constraints of existing DNA synthesis technology so that they can tackle the world’s most pressing challenges across healthcare, agriculture, manufacturing, and sustainability.
What is engineering biology and what are some of its applications?
Engineering biology is an exciting field that uses tools such as gene editing and machine learning to design or redesign biological components, in order to generate a desired outcome. It has far-reaching potential to help us with many of the challenges that humanity is facing, such as sustainability, climate change, healthcare and agriculture.
For example, engineering biology enabled the rapid development of COVID-19 vaccines using mRNA vaccine technology and is enabling the replacement of petrochemical fuels and plastics, as well as providing a more sustainable alternative for the synthesis of chemicals.
What is the role of synthetic DNA in engineering biology?
Synthetic DNA is fundamental to the Design-Build-Test-Learn (DBTL) cycle used in engineering biology. Achieving the desired outcome comes down to designing the optimum sequence within the “Design” phase, using computational approaches and in silico testing. Functional testing of that sequence is dependent on building that sequence through DNA synthesis and assembly technologies. The iterative nature of the DBTL cycle means that multiple rounds of DNA design and build are generally required before the desired outcome is achieved and doing this at scale is entirely dependent on being able to synthesize synthetic DNA rapidly and accurately.
What are some of the current challenges facing engineering biology?
One of the biggest challenges facing engineering biology is its ability to scale. We saw the power of engineering biology at scale during the COVID-19 pandemic when mRNA vaccines were developed in record time. However, these projects had unprecedented access to funding and other resources needed to achieve this. Engineering biology has the potential to solve many long-standing issues, but it needs to be able to scale efficiently to have maximum impact.
The development of sophisticated new AI tools allows scientists to redesign existing sequences and even design sequences that encode entirely new proteins; this allows them to explore the sequence space like never before and will contribute enormously to scaling engineering biology. However, testing these sequences requires rapid, accurate synthesis of gene-length DNA, and current technologies are not able to keep up, creating a bottleneck.
DNA synthesis has generally become a centralized service, provided by a small number of suppliers. This can lead to delays as this model is impacted by shipping times and costs, as well as the time needed to synthesize the DNA. These delays are more acute with longer sequences that require time-consuming post-synthesis workflows, and it can take weeks or months for long or complex sequences to be delivered.
What do you think is the biggest barrier to improving DNA synthesis?
The ability to generate accurate, long DNA is essential for the DNA synthesis bottleneck to be alleviated. Several factors make producing gene-length DNA challenging. Firstly, the efficiency of the elongation cycle, determined by the accuracy of nucleotide incorporation into the sequence, poses an increasing challenge as the length of the DNA sequence increases. For instance, with an elongation cycle efficiency of 99.5%, the theoretical yield of error-free sequences for a 120-base DNA fragment is 55%, the yield decreases to 37% at 200-bases, and increasing the DNA length to 1 kilobase, causes the yield to plummet to less than 1%.
Because of the errors incorporated during synthesis, longer sequences are assembled from purified shorter sequences. But even then, the time and cost associated with assembly and error correction pose an additional challenge. Better technology for the synthesis, assembly and error removal of long, accurate DNA will significantly reduce development timeframes in engineering biology.
How can technological developments address these challenges?
To address these challenges, two issues need to be solved: access to gene synthesis and error removal. A shift away from centralized services will allow scientists more control over gene synthesis and enable greater predictability and agility in their workflows, which will, in turn, allow faster iteration. Streamlined error removal will also speed up development programs by significantly decreasing the time taken for post-synthesis steps. Our approach aims to solve both challenges by developing a benchtop gene synthesis technology that incorporates thermally controlled, highly parallel DNA synthesis, staged assembly, and error removal directly on the surface of a semiconductor chip.
We use re-engineered DNA synthesis chemistry to allow for selective elongation at specific reaction sites via temperature-sensitive protecting groups at the sequence termini. DNA synthesis is controlled at thousands of independent reaction sites on a silicon chip, resulting in a microfluidic thermal array that allows for precise synthesis of DNA sequences in parallel. Our patented Binary Assembly® process then joins DNA strands with complementary sequences, taking advantage of the microfluidic environment and the charge on DNA molecules to selectively transfer DNA from synthesis sites to assembly sites on the silicon chip. After complementary strands are annealed, the assembly sites are heated to sequence-dependent temperatures that promote rapid dissociation of imperfect matches from the chip, thereby separating and removing error-containing sequences whilst maintaining those that are error free.
What impact will Evonetix’s technology have on the future of engineering biology?
The main use for our technology will be to reduce the time it takes to iterate researchers’ designs and extend the complexity of the designs that are possible. Currently, the design-build-test cycle is restrained by the lengthy timescales of the ‘build’ process. By putting DNA synthesis directly in the hands of the researcher, we give them the opportunity to move through the cycle much faster, which in turn will greatly impact the scalability of engineering biology.
This post was supported by Evonetix.