Ping! Genetically engineering bacteria with sonar capabilities
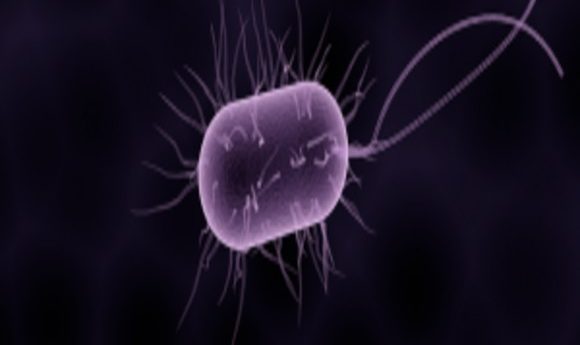
While many scientists are excited about the possibility of using genetically engineered bacteria to help treat a host of diseases, there’s one ongoing problem—it’s hard to determine just where these bacteria go once they enter the human body. Now, scientists have re-engineered bacteria so that they can be tracked by ultrasound.
We are coming to a new era: an age of genetic engineering—a time when scientists can, and do, alter bacteria and other types of cells to create new investigative, diagnostic, and therapeutic tools.
“There’s been a lot of great work taking cells, whether they are microbial or human cells, and engineering them to go into the body and carry out certain tasks,” said Mikhail Shapiro from the California Institute of Technology (CalTech). “The advantage of working with cells is that unlike a simple molecule, as we see in pharmacological agents, cells can do some really sophisticated things. They can go through the body, recognize molecular signals, and then perform logic and computation based on those signals that may lead to releasing therapeutic proteins, killing cells or pathogens, multiplying to make more of themselves, or self-destructing when they are no longer necessary. They are very sophisticated agents.”
Yet, despite their sophistication, there is one ongoing problem: it is hard to track bacteria in the body once those genetically engineered cells have been ingested or injected. Raymond Bourdeau, formerly a post-doctoral fellow in Shapiro’s lab and now a scientist at Codiak Biosciences, said that this remains a huge challenge for scientists and clinicians alike.
“Many studies have created cells to do very complex designed functions, but it doesn’t end up in the right spot so that it can do what it needs to do,” said Bourdeau. “The question then becomes how to know where these cells are going and what they are doing once they get there.”
From GFP to Sonar
In 2008, the late Roger Tsien, a biochemist at the University of California San Diego, took home the Nobel Prize in Chemistry with colleagues Osamu Shimomura and Martin Chalfie for the discovery and development of green fluorescent protein (GFP), a fluorescent protein that allows scientists to visualize cell signaling and gene expression. Tsien is one of Shapiro’s scientific “heroes” who inspired him to see if there might be a way to create a bio-inspired marker like GFP that responded not to light, but instead to some property that would be easier to visualize in the opacity of the human body.
“At some point, I learned about the existence of these protein nanostructures called gas vesicles in photosynthetic microbes called cyanobacteria,” Shapiro recalled. “The gas vesicles allow the cell to become buoyant in bodies of water so these microbes can float near the top to get enough light for photosynthesis. I immediately wondered if these nanostructures could scatter sound waves, making them able to be seen on ultrasound.”
Ultrasound technologies track high frequency sound waves—and ultrasound imaging is routinely used in hospitals and doctor’s offices to help visualize muscles, tendons, and internal organs for diagnostic purposes. To date, there are no known risks of using this visualizing technique on human patients. If these gas vesicles could be picked up by ultrasound, then bacteria genetically engineered to produce them should show up using this common visualization method, making it possible for scientists and clinicians to see those bacteria in the body the same way that Navy technicians can pick up a submarine in the ocean.
And sure enough, when Shapiro and his colleagues isolated the acoustic reporter genes that provide the proteins to build these nanostructures, they saw that they were able to produce ultrasound contrast (1,2).
“We know that the physics were there for them to work with ultrasound. But the open question was whether we could get the biology to work if we put those genes into a different species,” Shapiro said.
Re-engineering for Ultrasound
Transferring the genetic blueprint of gas vesicles from one bacteria to another turned out to be more difficult than transferring GFP across species. “There’s just a single protein that encodes for GFP,” Shapiro said. “In the case of these vesicles, there are a group of genes, these acoustic recorder genes, that produce the proteins that work together to assemble the gas vesicle structure. It’s bigger than a single protein—it’s a bunch of proteins that combine together. We learned that we needed 11 different genes to be expressed together and then function together in order to assemble the nanostructure.”
After several attempts, the team finally succeeded in transfering these genes from two types of cyanobacteria, Anabaena flosaquae and Bacillus megaterium, into E. coli, which is commonly used for microbial therapies. This winning combination allowed E. coli to assemble the sonar-bouncing gas vesicles on their own.
“We learned that it’s important to take some of these genes from organisms that are more compatible with E. coli so they will come together in the right way,” said Shapiro. “To make it work, we needed to combine two proteins from one species and nine proteins from another.”
According to Bourdeau, the end result is bacteria that can be visualized and located using ultrasound in the guts of mice—which is important for future human applications (3). “Ultrasound allows us to see deep into the body,” he explained. “We can maintain a precision and high resolution deep inside biological tissue. As we move forward and evaluate different technologies in engineered cells, especially in organisms like humans, having this technology gives synthetic biologists, basic scientists, clinicians, and the biotech community a new tool to use.”
Moving Towards Human Use
This study is one step towards what will hopefully be a long and fruitful road of research and development. Shapiro hopes that researchers will soon be able to express these acoustic reporter genes in bacteria that are being developed for specific therapeutic purposes— to help validate their efficacy and safety in human patients.
“I don’t see any reason why we couldn’t do this in humans,” said Shapiro. “And it’s important that we do. The things we want to learn about bacteria and health aren’t the kind of things you can learn in a dish. You have to study these phenomenon in an actual living, breathing organism to see how things are interacting with these bacteria. The spatial location of these microbes is important to understanding their function—and it’s our hope that these acoustic reporter genes will provide a new tool for scientists to understand the basic biology of how bacteria interact with mammalian hosts.”