PDX models: tips, tricks and trends in cancer research
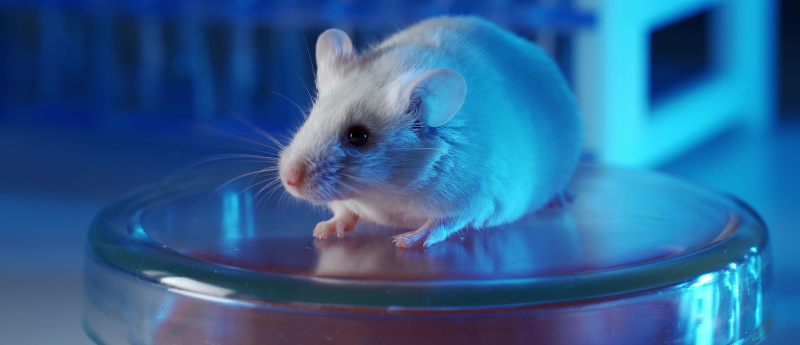
Alana Welm (left) is the Senior Director of Basic Science at the Huntsman Cancer Institute (University of Utah, UT, USA). Her lab studies breast cancer and its metastasis – the main cause of death for breast cancer patients – utilizing both genetically engineered mouse models and patient-derived models.
Here, she describes the utility of patient-derived xenograft (PDX) models in her work, the advantages they provide compared to organoids and traditional animal models and the challenges associated with their use.
What are PDX models and how do you establish them?
A xenograft is defined as the grafting of tissue from one species into an organism of another species. To use these in cancer studies, we take tumors directly from patients and grow them in mice, which gives us a whole animal model of the disease. This is especially important when you’re studying the complexities of cancer such as its ability to metastasize and spread to other organs; that’s currently impossible to study in culture models such as tissue culture or organoid models.
And what are the advantages of these models over traditional animal models?
A patient-derived xenograft also has the advantage that we can grow tumors from modern-day patients that are undergoing today’s treatment regimens, so we’re getting tumors that are resistant to current therapies. This makes them far more relevant than some of the old cancer cell lines, which came from patients many decades ago and might not reflect today’s treatment regimens. This is important for trying to find the next therapy because many of these tumors are resistant to our current treatments.
The other important advantage of these patient-derived models is that the tumors we’re studying reflect real human tumors, not just the treatments that those patients have received, but also the genomic composition of the tumors. When we generate tumors in a genetically engineered mouse model, we typically do that by expressing particular oncogenes and/or knocking out tumor suppressors that will create the tumor. However, that tumor is not as complex as it is in humans, where there may be hundreds or thousands of mutations that have accumulated during that patient’s tumor development. So, by studying tumors from patients, we get the genetic complexity of those tumors as well.
How can these models be utilized in an immuno-oncology setting?
One of the limitations of PDX models is that most of the time they’re growing in mice that are engineered to be immunocompromised. Of course, the reason for that is because we’re asking the mouse to host a tumor from a different species and if the immune system were fully competent it would reject the human tumor. Growing these tumors in an immunocompromised setting is a disadvantage as we cannot study the tumor-immune interactions that we know are important for tumor progression and response to therapies, especially immunotherapies.
To overcome this issue, people have tried to “humanize” the mouse immune system using hematopoietic stem cells from human patients or donors. We can implant these stem cells in the mouse at the same time as the tumor, enabling us to reconstitute the immune system of a human in the mice and produce a humanized PDX model.
This approach works well, and we have seen evidence that those immune cells can react to the tumor. For example, if you give an immunotherapy to those mice, we observe an effect on tumor growth and even tumor rejection. There are still limitations, however, because those human immune cells are now developing in the context of a foreign host and so they’re missing a lot of the signals that they would normally receive in a human. I would say that the immune system is there but not fully functional, so there’s a limitation in trying to study those tumor-immune interactions in a humanized PDX model.
Does the humanization of the mouse immune system impact the physiology of the mouse in any other ways?
It really depends on the method that one uses to establish the human immune system in the mouse. Immune systems are very complicated, and their job is to recognize foreign cells or other foreign antigens and kill them; if you take a mature human immune system and you put that into the mouse, it will recognize the mouse as foreign, leading to graft-versus-host disease.
This is typically avoided by implanting immature human immune cells – hematopoietic stem cells from umbilical cord blood or bone marrow – that are less likely to cause graft-versus-host disease. Once they’re established in the mouse, they’ll mature within the host and be somewhat tolerized to the mouse, but they’ll also be lacking some functionality because they didn’t develop in a human host.
However, if you have an immune system from one donor and a tumor derived from a different patient, then the cells are not matched. This may lead to a situation where the immune cells attack the tumor, not because they recognize it as abnormal cells growing in that individual, but because they recognize it as a tissue from a foreign organism. This confounds your results and clouds the conclusions that you can draw from your experiment.
To avoid this, you can match the donors for both the tumor and the immune system, known as an autologous transplant, but that is a lot more difficult and expensive. We can match according to human leukocyte antigen types, for example, just like one would for an organ transplant. There are different levels of matching, from very good matching to almost no matching and each time you’ll have different degrees of recognition of the tumor as self or as foreign.
Most of the time people don’t match at all, in part because the data has shown that the immune system doesn’t really reject the tumor even when it’s from a different individual. We’re not sure if that’s because the human immune cells developing in the mice are non-functional to some degree, or if it’s because the tumor has such an immune suppressive environment, which we know is a feature of tumors. It is all very complicated.
How do PDX models compare to organoid models in terms of complexity?
Maybe I should define organoid models first. In the cancer field, we can grow tumors in three-dimensional (3D) cultures in vitro. A key feature here is the matrix within which they develop, which mirrors the natural in vivo environment that a tumor would typically develop in. That’s called a tumor organoid, but it’s essentially a little tumor mass.
The cells in these models organize themselves very well relative to how they are organized in a tumor, but they differ from PDX models because they don’t have other body components around them; there are no blood vessels, immune cells or other stromal cell types in that culture after growing it for some time, it’s usually just pure tumor. This makes the model deficient in stromal components, including immune cells. People have tried to address this deficiency by co-culturing the tumor cells with immune cells, and fibroblasts, for example. These supporting stromal cells can infiltrate the organoids, surrounding the tumor cells to try to recreate the in vivo environment, but without the need to use an animal.
The lack of animal involvement is a clear advantage as it makes experiments more feasible, reduces time and cost and, of course, resolves the ethical issue of having to use animals. But there are disadvantages as well, particularly when you are studying metastasis, as there is no full system element to it. Studying metastasis, you’re trying to understand how a breast tumor ends up growing in a site like the brain or liver and how the tumor differs at these separate sites. We advocate for a combination of these models in order to best address the question at hand.
HUB tumor organoids for oncology drug development
This fact sheet explores how patient-derived organoids can capture the complexity of cancer and predict patient response to treatment in the clinic.
Have you seen a change in your field since the passing of The FDA Modernization Act 2.0?
That act removed the requirement to test all drugs in animal models prior to approval. However, I wouldn’t say that I’ve seen a great change in the field since this was passed. We were already moving in that direction, both due to a desire to use fewer animals in research but also to increase feasibility. Animal work is slow, expensive and resource intensive.
The first thing that had to happen was to show the fidelity of the organoid systems. PDX models have been around for a lot longer and we’ve validated them as good, but not perfect, models of cancer; the same had to be done with organoids. As the data accumulates to show that organoids do nicely model human tumors, people start to gravitate toward this new state-of-the-art method.
How would you choose between the use of these two models for different applications?
To answer the most pressing questions in cancer biology, the use of multiple model systems is the best way to go. I usually advocate for mechanistic studies to be conducted in organoids or other cell models, and then for more complex whole-body questions, like metastasis, a PDX model is more appropriate.
Drug treatment experiments are good to do in both organoids and PDX models, but the latter provide the additional advantage of assessing the tolerability of the drug, making sure that you can, for example, shrink a tumor at a dose of a drug that’s tolerated well by the animal. For immuno-oncology, I still think the best models are the genetically engineered models that have entire intact healthy immune systems.
Do you have any tips for best practice when implementing PDX models in your research?
I think best practice is to use orthotopic models, where you inject the tumor into the location of the mouse that matches that tumor. For example, for breast cancer, we put the tumor in the mouse mammary gland. Many people do subcutaneous injections because that makes the tumor easy to see and measure, but this is not the best environment for a brain tumor, for example, and we know that the environment really does affect the biology of the tumor. So, while the subcutaneous model is easier to study, it won’t be as relevant to the native human tumor.
Considering the host mouse is also important. The mice that we grow PDX in come from different genetic backgrounds and mostly the differences have to do with how immunocompromised the animal is. NOD/SCID gamma mice are currently the most immunosuppressed models that are widely available, so the take rate is higher, meaning the percentage of animals that will grow a tumor after it’s implanted is high. What’s more, the growth rate is higher, as is metastasis frequency. However, that might not model human disease very accurately so if you use a less immunosuppressed animal, such as NOD/SCID, which has functional natural killer immune cells, you can at least kind of recapitulate some of that immune biology. Depending on the question at hand you can pick between these models to select not only the best model, but the best practices for that model to address your question.
If there was one thing that you could ask for to improve PDX models what would it be?
I have to come back to immune competency. I wish we had a way to utilize PDX models in immune-competent hosts. Maybe that requires improvements in humanizing mouse immune systems or a completely different creative solution. I think that’s the genie that we need right now in this era of immuno-oncology.
Disclosure: The University of Utah may license PDX and organoid models to for-profit companies, which may result in tangible property royalties to the University and members of the labs that developed the models, including Dr. Welm’s lab.