A peek behind the paper: Megan Valentine on the 3D-printable device that allows cell monolayers to be dynamically compressed and imaged
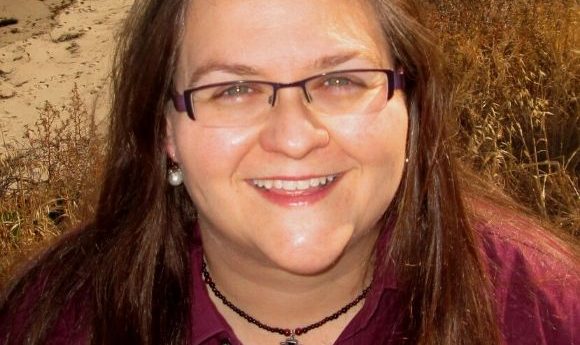
Take a look behind the scenes of a recent benchmark article published in BioTechniques entitled ‘3D-printable cell crowding device enables imaging of live cells in compression’, as we ask lead author Megan Valentine (University of California, Santa Barbara, CA, USA) about her new device that enables direct control of cell density in epithelial monolayers.
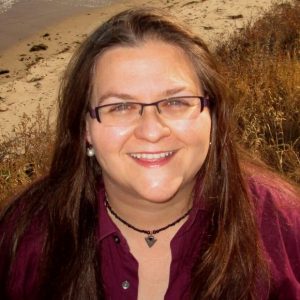
Megan Valentine is a Professor of Mechanical Engineering and Associate Director of the California NanoSystems Institute at the University of California, Santa Barbara (USA). She is an expert in the development of methods and tools that enable quantitative measurement of cellular forces and cytoskeletal mechanics. She engineers high-performance bioinspired materials that capture the impressive properties of living materials, including the ability to respond to stimuli, move and heal. She has received numerous awards including a Burroughs Wellcome Career Award, National Science Foundation CAREER Award and a Fulbright Scholar Award. She is a fellow of the American Physical Society.
Please can you give us a short summary of the recent benchmark article, ‘3D-printable cell crowding device enables imaging of live cells in compression’?
We designed and created a new device that allows cell monolayers to be dynamically compressed and imaged. This is challenging because it requires cells to be cultured and grown for hours to days on stretched membranes, which are then controllably relaxed, compressing the cells. To achieve this, we use 3D printing to create low-cost compression devices that can be placed in any cell incubator then transferred under tension to a microscope for imaging.
What led you to carry out this research?
I am very interested in the mechanisms by which cells in a monolayer sense their local density, how they decide whether to move or divide, and how individual cells are removed from a monolayer if the density gets too high. Unfortunately, there are very few tools that enable such studies, so we decided to design and build our own. I believe scientific innovations and discoveries should be accessible to the largest audience, so we have included schematics, design files and detailed instructions which will allow others to recreate and utilize these low-cost devices.
What were the key conclusions; what impact do you foresee this research having?
We demonstrated that our device can provide up to 30% strains in plane, and that cells can be dynamically compressed, leading to rapid increases in cell density as well as changes in cell shape. This is an exciting result, and we anticipate that our devices will enable many new studies of dynamic cellular responses to compression. We hope that by making these tools broadly available to the community, we can accelerate these important discoveries.
What work are you hoping to do next in this area?
I’m most interested in using these technologies to study the responses of cells to rapid changes in cell density. I aim to not only understand how cells sense and respond to crowding, but also to reveal the physical mechanisms by which single cells are selected for extrusion, how they are removed, and how the remaining cells in the monolayer seal the hole they leave behind. Understanding these mechanisms is critical to understanding how blood vessels grow and shrink, how cell barriers are maintained within tissues, and how such mechanisms fail in cancers and other diseases.