Divide and conquer: studying cell division in cancer evolution
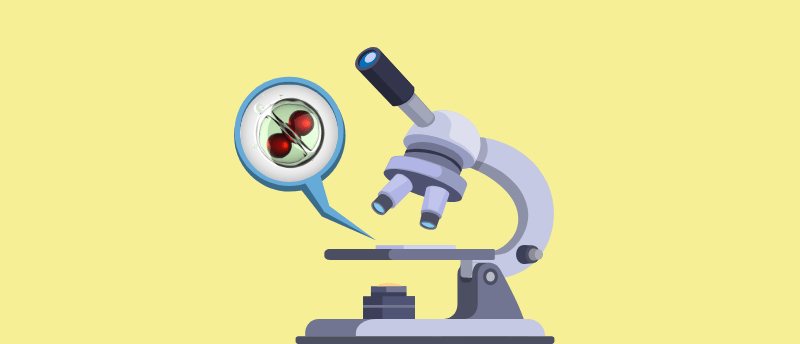
David Pellman (left) is a professor at the Dana-Farber Cancer Institute and Harvard Medical School (both MA, USA), who also has affiliations with Howard Hughes Medical Institute (MD, USA) and the Broad Institute (MA, USA). His focus is cell division and understanding how cell division errors generate catastrophic alterations to genomes, potentially leading to the evolution of cancer genomes.
In this interview from the American Association for Cancer Research (AACR; 5th–10th April; San Diego, CA, USA), where David received the G.H.A. Clowes Award for basic cancer research, we talk to David about the research that earned him this distinction, his technique – Look-Seq – and the big question that remains at the center of the cell division field.
Can you tell us about the research for which you’ve been awarded the G.H.A. Clowes Award for basic cancer research?
There are three key projects. In the early days of the lab, we worked on asymmetric cell division. That work was all done in yeast. In parallel with other teams, we worked out the pathway of how the mitotic spindle senses the polarity of cells and is positioned properly across the two dividing cells. That’s an important process for generating one stem cell and a more differentiated cell. It involves interesting things like the assembly of certain kinds of actin filaments by proteins that we worked on called formins.
The second project was resurrecting a 100-year-old idea that failing cytokinesis, doubling all the chromosomes and centrosomes could drive tumor development. In 2005, we published a paper where we did a simple and direct experiment to test that idea in a mouse, and we were able to show that whole-genome duplication can drive tumor development. Whole-genome duplication is common in organismal evolution; the extra sets of chromosomes are thought to provide fodder for evolutionary experimentation. It made sense in some ways that whole-genome duplication could drive the somatic evolution of tumors. Our experiment said it was possible but didn’t say how common it is. From genomic analysis by others, it is now clear that whole-genome duplication happens in about 40% of all human tumors at some point in their evolution.
The third point, which grew out of the work on whole-genome duplication, is that we worked out a mechanism to explain a recently discovered phenomenon in cancer called chromothripsis. It’s a neologism for chromosome shattering. Without going into all the details, when you have an event like whole-genome duplication that disorganizes mitosis, you can wind up not getting the chromosomes segregated properly so one or a few of them wind up in their own nuclear structure called a micronucleus. We found that you get massive DNA damage on the missegregated chromosome when it’s in a micronucleus, and that explains the isolated shattering of one or a few chromosomes in chromothripsis. We were able to test that directly because we developed a technique called Look-Seq, where we could combine long-term life-cell imaging with single cell whole genome sequencing. So, we could make standard cell biology manipulations and induce chromosome missegregation and micronucleation, watch that all happen, watch a surrogate occur for the acquisition of DNA damage, follow the cells through cell division, and find the damaged chromosome getting incorporated into daughter cell nuclei. Then we could use semi-robotically controlled microcapillaries to grab the individual cells from the culture and do complete whole-genome sequencing. This essentially recreated chromothripsis in the laboratory and showed that it could originate from these abnormal structures of the nucleus.
What techniques are essential to your lab’s work?
I think technologically, Look-Seq and variations that we’ve recently developed to study transcriptomes in single cells after identifying them by imaging is the most unique technology in the laboratory. We’re very focused on biological problems and trying to answer questions, and we try to do whatever is appropriate to address those questions. We use a range of approaches, but I have a background in genetics and cell biology, so I would say the core is molecular genetics and cell biology.
Do you have any key tips for best practice when using Look-Seq?
We’re going to write some methods papers to more widely disseminate how to use the technique. One of the trickiest parts is the data analysis, which is a bit of a moving target. I’ve been fortunate to have had a marvelous collaboration with a former trainee, Cheng-Zhong Zhang (Harvard Medical School, MA, USA), who’s now an independent faculty member in the data science department at Dana-Farber Cancer Institute and the pathology department at Harvard Medical School (both MA, USA).
I would say that there’s an element of luck involved in finding the right people for this project– you need a computational biologist who has a good understanding of biology. The technology is in principle fairly straightforward. It’s similar to a correlative light and electron microscopy (EM) experiment, except, instead of the EM, you use DNA or sequencing or transcriptome analysis to increase the resolving power of the light microscope,.
Right now, I think one of the major limitations of doing this is that you can only study events that happen frequently because it’s laborious to isolate the cells of interest. There are all sorts of interesting technologies being developed, for example, photo marking cells and then isolating them after the imaging by FACS sorting. The issue for us is that when we see something interesting and specific that we want to know more about, we want to have a high depth of sequencing and extensive analysis of the genomes that we’re looking at. The methods that are geared toward digital karyotyping resolutions in the Mb to 200 Kb are not suitable for what we’re doing now. Until recently, we haven’t been pressed to try to improve that aspect of the technology because the rate-limiting aspect was the cost of a 30x genome sequence on a single cell. This has now dropped to around US $350 per cell. Now it’s more feasible to go for higher throughput.
If you could ask for one thing to improve your understanding of cell division, what would it be?
One question that I’m fascinated by is how you assemble a normal nucleus. One would think this would be better understood than it is. Abnormal structures of the nucleus drive a lot of the genome instability that occurs in cancer, and the starting point to understand this is to understand in detail how the normal process works. I’m interested in why some tumors are haploid or near haploid whereas others are near tetraploid. I think in general the field has made good strides in understanding basic mechanistic processes. But how that impacts specific tumors and why there is such incredible variation in how these different events manifest in one tumor versus another is not well understood.
The opinions expressed in this interview are those of the interviewee and do not necessarily reflect the views of BioTechniques or Taylor & Francis Group.