Everything you should know about CRISPR/Cas9
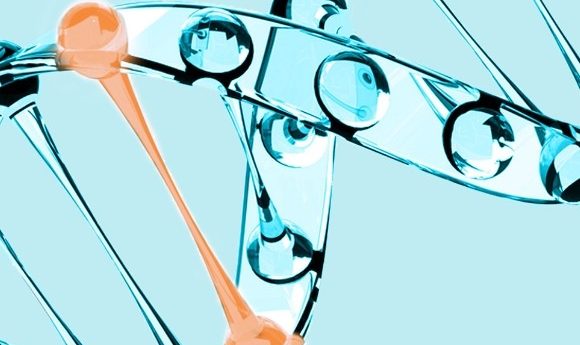
Eric Kmiec, PhD, Director of the Gene Editing Institute at the Helen F. Graham Cancer Center & Research Institute of Christiana Care Health System, discusses its use of CRISPR to edit DNA outside of the cell for the first time, the hype surrounding CRISPR and its initiative to bring gene editing experiments into colleges and high school laboratories.
Please can you introduce yourself and tell us about your background?
My scientific career began as an undergraduate at Rutgers University (NJ, USA). I then received a Masters from Southern Illinois University (IL, USA) and eventually a PhD at the University of Florida Medical School (FL, USA).
During my PhD, I began working on homologous recombination – the overall process that’s used in gene editing – now known as homology directed repair. I studied how this type of recombination event directs evolution and the implications for that. I then began a long career focusing on how the proteins and enzymes actually acted to yield DNA and to pair chromosomes together.
My first faculty appointment was at the University of California, Davis (CA, USA), where I continued in this work and eventually found my way to the University of Delaware (DE, USA), where I spent 12 years as a tenured professor in the Department of Biology.
A few years ago, after meeting with the medical director of the Helen F. Graham Cancer Center & Research Institute at Christiana Care Health System (DE, USA), one of the nation’s largest community-based health systems, I was convinced that the only way to truly do translational research in Delaware would be to embed a gene editing program within a community cancer center.
Delaware does not have its own medical school – it’s small. So, we utilize Thomas Jefferson University (PA, USA) as the medical school for Delaware. There aren’t a lot of impactful clinical opportunities to carry out translational research at the University of Delaware, so I lifted my 13 person lab and moved to the cancer center three years ago. I retained a faculty appointment at the University of Delaware and have also now acquired a faculty appointment at The Wistar Institute (PA, USA). Right now, there are 15 people in the lab and they range from senior research associates to post docs to graduate students.
What brought you to the field of gene editing?
I was working peripherally in this area in a time I usually call BC – or ‘before CRISPR’. The available tools at that time were not as elegant or, frankly, as good as they are now. My transition to the field came about because the perception of what we refer to as gene editing has always been about correcting or repairing genes. The gene editing reaction uses the same enzymes and regulatory factors that pair chromosomes and are involved in DNA replication or recombination activities.
So, while we continue to keep a strong interest in the enzymology of homologous recombination, there’s just a natural fit for transitioning to translational medicine, applying gene editing to human cells. Our knowledge of how strands of DNA come together and how the cell deals with DNA pairing reactions is at the center of the entire gene editing reaction. Clinical, agricultural and pharmacological applications are natural extensions of this fundamental work.
The Gene Editing Institute recently published a paper detailing its novel use of CRISPR to edit DNA outside of the cell. Please can you tell us some more about this?
We are dedicated to finding out how CRISPR gene editing works at the enzymatic and molecular level by studying the mechanism of action and the regulation of gene editing inside the cell. Laboratories, like ours, are treating primary cells and animal models with gene editing tools, and in these reaction protocols, the editing tools are introduced into cells, then 24 or 48 hours later, you measure the outcome. In these experiments, you don’t really know what’s happened inside the cell, you are just happy it worked or disappointed it didn’t.
We can guess and, of course, there are sophisticated techniques to do that, but our approach has always been to try and dig out the guts of the reaction and understand its internal workings. We developed a system where we can actually utilize CRISPR and a cell-free extract to carry out exactly the same reactions that are done inside a cell and get essentially the same outcome whether its deletion, insertion or replacement of genes. In my opinion, this work will lead to a fundamental understanding of what makes this whole process work and that will uncover the best approaches to enable gene editing in primary cells to work more efficiently.
From purely a reaction standpoint, the CRISPR/Cas9 complex enters the cell and executes DNA breakage signaling where the edit is to take place, but, in the end, the endogenous protein activity in the cell edits the DNA. Many studies have indicated that breaking the DNA followed by resection of the broken ends is more efficient than the insertion of a piece of corrected DNA that is designated to rescue a genetic mutation. We call this the CRISPR imbalance and it’s at the heart of many investigations aimed at improving precision and efficiency. We approach this problem by breaking the enzymatic reactions down into fundamental pieces and putting them back together one by one so that we can understand what causes this genetic imbalance.
Using this experimental strategy, you can manipulate the extract by depleting a particular enzyme thereby defining its role. It then becomes more of a reduction to practice in a biochemical sense. As nucleic acid enzymologists, this is really exciting because we want to optimize the gene editing reaction for repairing genes, not just disabling them.
We recently published this work in the CRISPR Journal and one of the reviewers of our paper said they wished there were more papers like ours. Frankly, so do I. Everyone is racing toward therapy and we’re their biggest fans, but going too fast can sometimes lead to overpromising outcomes. I believe that this technology is so extraordinary that it will survive any excess hype that naturally accompanies big discoveries.
Knowing how it works and potentially being able to tweak it a little bit is going to be where major improvements are made. As more clinically oriented scientists get their hands on these regulatory factors and are able to manipulate their activity, it is likely that impactful therapies for genetic disorders will begin to emerge with regularity.
Which techniques do you use in gene editing and what are the pros and cons of these?
We did a lot of early work with ssDNA alone when we didn’t have CRISPR, so we employed donor DNA molecules known as oligonucleotides to carry out gene editing. This was successful and there were a number of groups using this approach, but we all found that the frequency with which single bases were repaired in the human chromosome was at a level that would not be clinically relevant.
When programmable nucleases appeared on the scene, that frequency was elevated quite significantly. Recently, many laboratories have moved to utilize adeno-associated viruses as the source of a donor DNA in gene editing reactions aimed at repairing a genetic mutation. Viral DNA overexpression is helpful to this process, but if there’s a red flag in that approach, it’s that high levels of ssDNA in a cell will likely induce several regulatory proteins that respond to cellular stress.
One of these is p53, a well-known marker for oncogenesis. Eight years ago, we reported that p53 is induced in gene editing reactions where anticancer drugs were used to execute DNA breakage prior to repair. So, I wouldn’t be surprised if overloading a cell can lead to some adverse responses that may or may not impact the outcome of gene editing in human cells.
We used to work with TALENs but we don’t use them anymore. They’re a wonderful reagent, but cumbersome to work with. TALENs are probably more specific than CRISPR, especially when it comes to the current concern of offsite mutagenesis, but this is a tough problem to overcome because you’re essentially trying to prove a negative – you’re trying to prove that something didn’t happen.
I don’t see that as a major block to the implementation of CRISPR-directed gene editing in human cells, although skeptics have a significant voice in this field – and we want to hear from them. We’ve now moved to using Cas12a (Cpf1) instead of Cas9, and other workers are utilizing Cas9 nucleases from other sources. New categories of CRISPR are coming fast, many of which have interesting characteristics.
One emerging concern in the field is that much of the technology developed by major groups is now licensed to, and owned by, a small number of biotechnology companies. While they have, by and large, allowed workers to use them for research purposes without fees, accelerating these discoveries toward clinical application will undoubtedly require license agreements and legalities that will have the unintended consequences of restricting innovation.
About 20 years ago I lived through the promises of gene therapy cures being right around the corner, so now I am a little more skeptical as well as being a bit concerned that overpromising therapeutic outcomes will end up with the same fate. Many of the same laboratories that dominated the early days of viral gene therapy are now heavily engaged in the gene editing technology.
What are the key goals of the gene editing institute?
I see this field as being more than just developing therapeutic strategies for CRISPR. So, I started the Gene Editing Institute with four basic missions.
The first is developing CRISPR as an augmentative therapy for various types of cancer, including lung cancer and melanoma. We are fortunate to be integrated inside a community cancer center. Nationwide, over 85 percent of patients get treated for cancer in community cancer centers, as is the case in Delaware. In addition, these patients are mostly naïve in terms of drug therapy, meaning that they have not been previously treated with a drug in a clinical trial. Our accrual rate to cancer clinical trials is outstanding and I feel strongly that our cancer patients, some in economically challenged situations, deserve to have the option of frontline state-of-the-art therapies.
We have an extremely strong, integrated team including patient advocates, genetic counselors, oncologists and scientists working together to develop effective yet safe protocols for these patients. We are working hard to develop the center as a place where all cancer patients can be treated with the latest therapies. This mission also includes research into the mechanism of action and the regulatory circuitry surrounding human gene editing. To carry out these studies, we’ve had strong support from the NIH.
The second mission is education. I’ve been in this field for a long time, so I’ve lived through the dark ages and now the enlightenment has come. We were able to develop a series of reporter gene systems that helped us understand the mechanism of single agent gene editing. As CRISPR emerged, I thought that one of these systems would be valuable as a teaching tool for a laboratory exercise in gene editing.
So, we launched and developed that idea and won a National Science Foundation award to develop a full curriculum for instruction in gene editing technologies for four-year colleges, community colleges and even high schools. There are plenty of tutorials online, but this is hands on. You can’t have a sophisticated experiment in high schools or colleges or community colleges because they don’t always have the equipment to carry it out. We therefore developed a strategy that’s quite similar to the experiment we published in the CRISPR Journal for these students. They can get an answer from the experiment in 16 hours, which is great, as materials, timing and the students’ attention span won’t stretch beyond an activity that could potentially take 2-3 weeks.
Our third mission is a simple one. We continue to act as a core facility for research labs around the country, and some overseas, that want to use CRISPR but haven’t learned how to do it or how to make it. We provide the tools and a charge-back method for them to use in their research.
The fourth mission is partnership. We have a couple of signed agreements and we’re licensing the technology to a couple of biotechnology companies. One of these partnerships has been catalyzed by a grant from the US-Israel Binational Industrial Research and Development Foundation.
We have an excellent relationship with the Israeli biotechnology company, NovellusDx, with whom we are working to improve the precision and efficiency of its well-established cancer diagnostic assay. They are an outstanding company and the scientific strategies surrounding the use of gene editing in cancer diagnostics is just beginning.
NovellusDx remains an extremely innovative leader in this field and has licensed our part of its diagnostic assay directly from Christiana Care. This project is particularly rewarding because it demonstrates the ultimate bench-to-bedside research paradigm. The reaction improvement is essentially the one that we jointly published with scientists from NovellusDx, and we are continuing to utilize it to understand the basic mechanism while NovellusDx is implementing it in a commercial product.
Another key partnership is with The Wistar Institute in Philadelphia (PA, USA). We provide them with the gene editing tools for researchers involved in vaccine development, cancer research and immunotherapy. This relationship allows us to interact on a daily basis with top-line, well-funded research laboratories and the flow of information is bidirectional. They are making us better scientists and I believe we are developing new approaches for their own research.
It’s a really exciting time for gene editing, what exciting developments do you think the future holds?
For any genetic molecular translational approach, your first thoughts go immediately to therapy. A number of my colleagues have said that we’ll be able to cure all genetic diseases. I’m not sure if we’re going to get to that point, but helping people alleviate suffering and pain with a genetic tool is a great goal. I think the future will bring established FDA-approved CRISPR gene editing treatments for both cancer and genetic diseases, such as sickle cell anemia and maybe even cystic fibrosis.
Therapy always comes to the top because it directly affects people’s lives. However, there’s also remarkable work being done in agriculture and organ transplantation where pigs are being re-engineering to knock out endogenous viruses that can affect transplantation.
A lot of the work we do for academic colleagues around the country centers on knocking out one, two or three genes in a cell line. The ease with which you can create modified cell lines with a trained operator is quite extraordinary. From my perspective, pharmacogenomics has already been impacted quite dramatically and I think drug development and drug discovery will also be part of it.
I believe cancer will be an excellent target for CRISPR as, in most cases, you’re not trying to repair a gene. You’re trying to destroy oncogenes or genes that are contributing to resistance to chemotherapy or immunotherapy. Cancer is a great target because it’s asking CRISPR/Cas9 to do exactly what it does in a bacterial cell and that’s to destroy DNA, thereby allowing the tumor cells to be more susceptible to standard treatments.
One of the most extraordinary aspects of CRISPR-directed gene editing is the broad impact it has had on so many disciplines in the public platform, which has enabled important discussions about its use. There have been some new papers published using CRISPR as a diagnostic for HPV as well as articles about a home beer brewing kit. It seems at this point that the only thing CRISPR won’t be able to do is open your refrigerator door!