The evolution of CRISPR
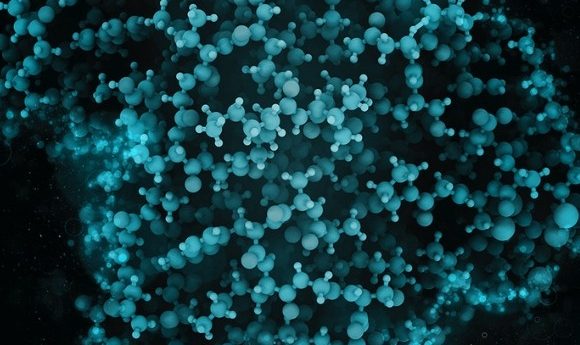
Ben Kleinstiver has been working with genome editing technologies since the time BC (before CRISPR). Here he explains why CRISPR accelerated in popularity so quickly, and how his lab is working to produce better CRISPR enzymes.
Ben Kleinstiver is a biochemist and genome editor whose interests include protein engineering and translating technologies into molecular medicines. He received his PhD in Biochemistry from the Western University (ON, Canada), and completed his postdoctoral studies at Massachusetts General Hospital (MGH) and Harvard Medical School (both MA, USA). Within the Center for Genomic Medicine at MGH, the Kleinstiver Laboratory seeks to address limitations of CRISPR genome editing technologies while solving important research questions at the forefront of the genome editing field.
Please can you provide an overview of your background with genome editing technologies?
Prior to the broad adoption of CRISPR technologies, there were several other genome editing platforms that researchers used to manipulate genomes. Some of the first technologies used in laboratories were proteins called homing endonucleases, which are enzymes that play a role in genome evolution. My PhD research focused on studying these proteins as naturally occurring proteins and also adapting them for genome editing.
Another major gene editing platform that researchers coalesced around in the late 1990s up to early 2011 were these proteins called zinc finger nucleases (ZFNs). ZFNs were the genome editing platform of choice for a long period of time. I worked with ZFNs for a short time during my PhD and then also studied another family of genome editing enzymes called transcription activator-like effector nucleases (TALENs). These are really intriguing proteins that became rapidly popular in 2011/2012, similar to what happened with CRISPR a few years later. TALENs were featured in the community for just a few years because gene editing with CRISPR was described shortly thereafter; CRISPR nucleases are RNA programmed, hence, researchers adopted CRISPR as a genome editing platform and never really looked back.
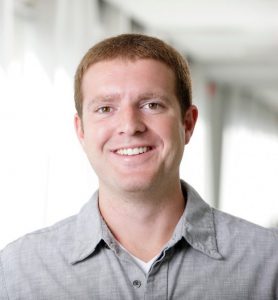
How have you seen CRISPR evolve and adapt since the beginning?
People were still able to edit genomes pre-CRISPR, just at a smaller scale and at a different pace. If you go into PubMed and do a search for ZFNs to see how many citations per year are attributed to that genome editing platform, there’s probably tens or hundreds per year at most. If you do a search for CRISPR, you’ll see a different trajectory of thousands of citations per year. It just speaks to how readily adopted the technology was and how transformative it has been for people around the world.
It really is trivial to re-design CRISPR to a new site. When people were using TALENs or ZFNs, they mostly had to buy these from companies with a cost in the US$10,000–20,000 range, which for most labs is completely prohibitive. The adaptation of CRISPR changed things because you can build a custom re-targeted gRNA for just a few dollars in the lab, which removed the cost and effort barriers that were associated with other technologies.
What research are you currently focusing on in your lab?
During my PhD, my research focused a lot on structure/function relationships, trying to study homing endonucleases. During my post-doc, to engineer and evolve new functionalities into CRISPR proteins, we adapted some of the systems that I optimized during my PhD. In my lab currently, we are now thinking about new methods of protein engineering to build better CRISPR enzymes.
When we engineer proteins, there are a handful of properties that we focus on. Firstly, you want CRISPR enzymes to be targetable, meaning you can pick nearly any specific sequence that you want to edit. The PAM sequence that a given CRISPR enzyme recognizes might prevent you from targeting certain sites in the genome, so we’ve engineered proteins that can recognize new sequences meaning that they can target more frequently and more freely. This allows researchers to pursue applications that they couldn’t before because they weren’t able to target the correct site.
Secondly, you want CRISPR systems to be safe, such that they don’t cut off-target sites. Genome editing technologies that are able to discriminate against other sites in the genome (that look a lot like the intended site) have major advantages in terms of safety and specificity. Since not all CRISPR enzymes naturally have highly specific targeting profiles, we can engineer new proteins that have improved specificity. This means that they can still cut at the intended site but can no longer cut at other sites in the genome where DNA cleavage would be undesirable.
More generally, we utilize protein engineering methods to impart desirable properties into naturally-occurring tools, taking what nature has given us and evolving versions of these enzymes that are more ideally suited for research applications.
Do you collaborate a lot with different research labs both outside or inside your institution and do you see benefits to that within CRISPR research?
My laboratory is in the Center for Genomic Medicine at MGH, where there are lots of research groups that have expertise and interests in disease genetics and biology. We are therefore collaborating with several groups to assess genome editing approaches to treat different monogenic diseases – which makes them fairly attractive targets for genome editing because we have high confidence that if we fix the mutation, it’s likely to correct that patient’s disease.
Outside of the institution, we have many ongoing collaborations where we have been able to share our engineered technologies with the research community, to both utilize and learn about the proteins we have built. These collaborations help us understand their strengths and weaknesses and really motivate further design, innovation, and improvement.
Working with people both in the research community and the preclinical disease research space, we get a nice sense of what the remaining needs of the technology are and that allows us to think of approaches to make better, safer, more useful enzymes. I think that really drives the innovation within my group in terms of making more broadly useful enzymes.
There are challenges with the development and use of CRISPR. How are these being overcome?
One of the major new frontiers of translating CRISPR into a therapeutic is the issue of immunogenicity; these are bacterial derived proteins being delivered into a human, and it has been shown that they can cause immune reaction. Another challenge is delivery; how do you get the genome editing technologies to the tissue or cell type of interest? Ideally, you want to deliver the genome editing technology only to the cells that you want to edit because then you can avoid tissue-specific off-target effects.
For these and other challenges, because the technologies are so accessible there are many researchers working on developing solutions. I don’t think that any of these challenges are insurmountable, it just may take time to develop creative and clever solutions.
Regarding using CRISPR for human therapeutics, to what extent do you think that’s going to happen in the future?
There are thousands of diseases that are in principle addressable by genome editing technologies. Some of the challenges, such as delivery and immune considerations, will play a role in how quickly the technologies can be adapted for different diseases. In principle, the reach for these technologies is very broad. Approaching your question from a very optimistic point of view, it is a very exciting time because we are able to edit the genome specifically and safely.
Right now, there are a handful of trials being run by therapeutic companies and these are important as proof-of-concept in terms of the efficacy, safety and specificity of the tools. The results and progress of these trials will be really informative, and should these trials show promise, they will pave the path for the much broader therapeutic adoption of CRISPR technologies.
In terms of your research and CRISPR tools in general, what do you see happening in 10 years time?
If you had a CRISPR crystal-ball, this would be an easier question to answer; however, the potential is tremendous. In the past few years there have been amazing functionalities added to ‘the genome editing tool box’ and I imagine there will still be several additional functionalities added in the near future. One very interesting and evolving area of research is the discovery of new enzymes. While the field has settled on just a couple of different CRISPR enzymes that have proven to be very effective and useful, I think we’ve just scratched the surface. Regardless of whether it’s CRISPR 1.0, 2.0, or what lies ahead, I am very optimistic that the technology will continue to make a big impact both in biomedical research and therapeutics.