Computer models nanoparticle–protein interactions
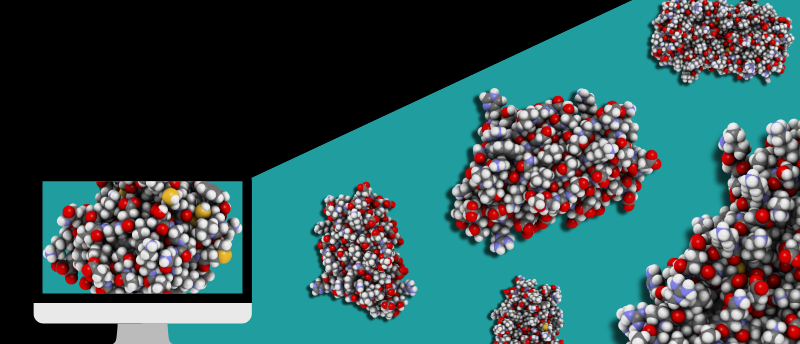
A new machine learning model, NeCLAS, can determine whether and how a protein and nanoparticle will bind, which could revolutionize drug discovery and design processes.
Researchers at the University of Michigan (MI, USA) have trained an AI model, named NeCLAS, to recognize protein structures and their binding sites and find the nanoparticles that might be able to interact with them. It also assesses how two molecules, either protein–nanoparticle, protein–protein or nanoparticle–nanoparticle, might interact and predict alternative binding sites to the known ones already encoded within the model. This technology could make designing drugs more efficient, allowing researchers to combat new and changing bacterial and viral threats.
This new model expands on what existing models are already accomplishing. For example, AlphaFold – an AI tool for predicting a protein’s 3D shape – lacks the ability to determine how the predicted 3D protein structures interact with other proteins as well as nanoparticles.
“That’s where NeCLAS comes in,” remarked Jacob Saldinger, first author of the study. “It goes beyond AlphaFold by showing how nanostructures will interact with one another, and it’s not limited to proteins. This enables researchers to understand the potential applications of nanoparticles and optimize their designs.”
Studying protein–ligand binding with Raman spectroscopy
A low-cost add-on to existing Raman spectroscopy systems measures protein–ligand binding at physiologically relevant conditions in real time.
Additionally, NeCLAS was trained to consider smaller molecules than other AI tools, which often consider amino acids as the smallest unit, allowing the model to explore small-scale interactions with more flexibility. It is also a more general model, receiving a smaller training dataset than existing prediction models.
The team went on to test NeCLAS on three case studies with the aim to determine: the molecules involved in creating molecular tweezers to halt protein aggregation in the brain, how graphene quantum dots break up a biofilm produced by a bacterium and whether water would cause these graphene quantum dots to disperse.
In future, the team plans to explore how this type of model could be used to predict how biofilms and microorganisms, such as viruses, interact with proteins, potentially streamlining the process of drug discovery and design in the years to come.
“In my ideal scenario, 20 or 30 years from now, I would like—given any superbug—to be able to quickly produce the best nanoparticles that can treat it,” concluded corresponding author Angela Violi.