CRISPR gene editing in large animals: applications, challenges and potential
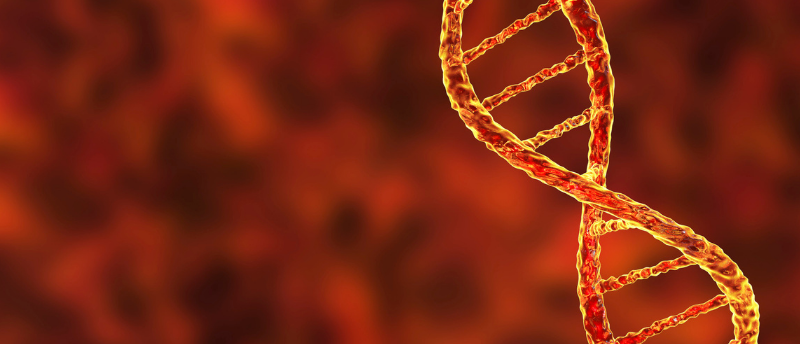
From model development to testing innovative gene editing-based therapeutic approaches, Lobna Elkhadragy explains how CRISPR gene editing in large animals offers exciting opportunities in biomedical research.
What are current and promising future applications of CRISPR in large animals?
CRISPR offers countless exciting opportunities and applications in biomedical research utilizing large animals, as well as for enhancing livestock production. By precise genome editing that mimics disease-causing mutations, CRISPR has been used to generate numerous large animal models of diseases. These models enable study of disease mechanisms and testing of therapeutic interventions. Furthermore, large animals have been used for testing CRISPR-based therapeutic approaches for diseases including muscular dystrophy1, sickle cell disease and eye disease. The success of some of these CRISPR therapeutic studies in large animal models provided proof-of-concept and was followed by human clinical trials.
One of the promising applications of CRISPR in large animals is the production of xenotransplantation organs and tissues that have less potential for immune rejection and are safer for humans. This application has a great value given the scarcity of human organs needed for transplantation. There is also growing interest in applying CRISPR to enhance livestock production by improving productive and fitness traits in livestock, conferring animal resistance to infectious and transmissible diseases, and suppressing other species considered as pests for livestock. Overall, the CRISPR revolution offers exciting promises across several disciplines.
What are the advantages and limitations of CRISPR research in large animals as compared to rodents?
Applying CRISPR in biomedical research employing large animals such as non-human primates, pigs, and dogs provides several advantages. Compared to rodents, large animals share greater similarities with humans in physiology, anatomy, size, immunity and metabolism. These similarities make them excellent models for human diseases, ideal organ donors for xenotransplantation and better predictors of therapeutic efficacy in humans.
Importantly, large animals have a considerably longer lifespan which enables evaluation of the safety and efficacy of CRISPR gene editing therapeutics over longer timespans. These long-term studies are critical for determining the safety of CRISPR therapeutics prior to human application. On the other hand, short-lived small animal models, such as mice and rats, cannot address secondary pathologies that may arise years after a gene editing treatment. Also, being closer in size to humans than rodents, large animal models allow testing clinical dosing regimens that are more relevant to humans. Using these clinically relevant CRISPR doses in large animals further serves to assess the feasibility of large-scale manufacturing of CRISPR reagents.
However, large animal studies require specialized veterinary staff and facilities and are much more costly compared to studies in small animals. This makes initial testing in smaller animals that are more accessible and cost effective an essential first step, followed by large animal studies that can bridge the gap between small animal models and clinical studies.
What are options for delivery of CRISPR therapeutics in large animals?
Both viral vectors and non-viral methods are used for in vivo delivery of CRISPR in large animals. Adeno-associated viruses (AAV) are the most commonly used viral vectors for in vivo genome editing. These vectors can deliver DNA encoding Cas9 and guide RNAs (gRNAs) into specific tissues of the body based on AAV tissue tropism. Using tissue specific promoters to drive the expression of Cas9 can further increase tissue specificity. Non-viral delivery methods include nanoparticle-based approaches, such as lipid nanoparticles, that encapsulate messenger RNA (mRNA) encoding Cas9 and gRNA.
CRISPR is a key element of many lab scientists’ toolkits. In addition to its application in basic research, CRISPR is also being applied ass a diagnostic and therapeutic tool, and is generating invaluable insights for drug discovery.
What are the biggest challenges associated with in vivo CRISPR editing in large animals?
In vitro and ex vivo CRISPR approaches have already been employed with huge success in large animals. However, there is slower progress with in vivo CRISPR editing in large animals due to factors including challenges of efficient delivery of CRISPR components, challenges of targeting specific cell types, vector-associated immune response and off-target effects. When ex vivo approaches are used, edited cells that do not have off-target alterations can be screened and selected prior to cell re-injection.
In vivo delivery of CRISPR components by AAV is challenging due to the large size of Streptococcus pyogenes derived Cas9 (SpCas9), while AAVs have limited genetic packaging capacities. Strategies that have been used to overcome this issue include using smaller versions of Cas9, such as Staphylococcus aureus derived Cas9 (SaCas9), and using dual AAV vector system expressing intein-split Cas9. Another approach was the generation of Cre-inducible Cas9 pigs, which allow in vivo gene editing by delivery of Cre recombinase and gRNAs. However, this approach requires the development of genetically engineered animals, a process that is time-consuming and expensive in large animals. Also, this approach results in permanent expression of Cas9 in cells exposed to Cre recombinase, which could result in DNA damage, genomic instability, improper gene editing and deleterious cellular effects.
Due to the challenges associated with efficient delivery of CRISPR components in large animals, optimal applications would be restricted to those not requiring complete gene editing in the target organ. Examples of these applications include cases where the edited cells have a selective growth advantage or where a small level of restoration of protein expression is sufficient for phenotype change.
How is in vivo CRISPR editing being used as a therapeutic in large animals currently and what could the potential of this be?
In vivo CRISPR editing holds great promise for the treatment of diseases via a single injection. This approach is particularly feasible for treatment of monogenic diseases that require at least a partial restoration of a defected protein. Large animal models have been used to demonstrate the utility of in vivo CRISPR editing of somatic cells as a therapeutic approach, paving the path to CRISPR clinical utility. For example, in dog and pig models of muscular dystrophy1, systemic delivery of AAV expressing CRISPR components rescued functional expression of dystrophin and ameliorated the disease.
As large animal models do not exist for all diseases, several studies have investigated efficient in vivo CRISPR editing in normal animals. For example, systemic administration of lipid nanoparticles carrying CRISPR in non-human primates resulted in successful and durable knockout of the target protein transthyretin. In another study, subretinal injection of CRISPR components in non-human primates resulted in the anticipated edit of the target gene CEP290 at sufficient levels. CRISPR base editors have also been tested in vivo in large animals. For example, a recent study delivered base editors in vivo using lipid nanoparticles in non-human primates, leading to efficient reduction in blood levels of PCSK9 and low-density lipoprotein cholesterol. Together, such studies provide proof-of-concept for efficacious editing in vivo.
How far are we from a CRISPR therapeutic approved for use in humans and what steps need to occur for that to happen?
Based on the quickly accumulating evidence of successful in vivo CRISPR editing approaches in animal studies, I believe that we are close to approved CRISPR approaches that cure or alleviate a variety of human diseases. Indeed, several current clinical trials investigate the utility of CRISPR therapeutic approaches. These include ex vivo CRISPR therapeutics for cancer (by editing T cells) and blood diseases (beta thalassemia and sickle cell disease) and in vivo CRISPR therapeutics for Leber congenital amaurosis2 and transthyretin amyloidosis3. All current CRISPR clinical trials are based on somatic cell editing that do not result in DNA changes being passed to future generations. Embryonic cell editing, however, represents a major ethical concern.
For successful utilization of gene editing therapeutic strategies, further studies are needed and several challenges have to be overcome. Studies needed include rigorous evaluations to fine-tune vector dosing, efficacy, cell/organ targeting specificity, immunogenicity and long-term safety of novel gene editing therapeutics. The cost of clinical development and regulatory approval of CRISPR therapeutics might also be a challenge, especially for rare mutations/diseases that require personalized strategies. Despite these challenges and need for further studies, we are witnessing rapid and great advances in CRISPR applications, and in the near future CRISPR-based therapeutics would alter current treatment paradigms.
1 Duchenne muscular dystrophy, a muscular degenerative disease caused by mutations in the dystrophin gene causing frameshifts or loss of production of functional dystrophin.
2 Leber congenital amaurosis type 10, an inherited retinal degeneration which causes blindness
3 transthyretin amyloidosis, a life-threatening disease characterized by accumulation of misfolded transthyretin protein.
About the interviewee
Lobna Elkhadragy is a molecular biologist and pharmacologist whose research interests include the development of liver cancer models and novel therapeutic approaches. She received her BSc in Pharmaceutical Sciences and MSc in Pharmacology and Toxicology at Cairo University (Egypt) and received her PhD in Biomedical Sciences from Wright State University (OH, USA). She is currently a postdoctoral researcher in the lab of Ron Gaba and Kyle Schachtschneider at the University of Illinois at Chicago (IL, USA). Using CRISPR gene editing, she is working on the development of precision large animal models of liver cancer, including porcine and rabbit models, to serve as a platform for translational testing of innovative therapies, diagnostic and imaging modalities.
The opinions expressed are those of the interviewee and do not necessarily reflect the views of BioTechniques or Future Science Group.