For single-cell protein and RNA analysis, go FISH-Flow!
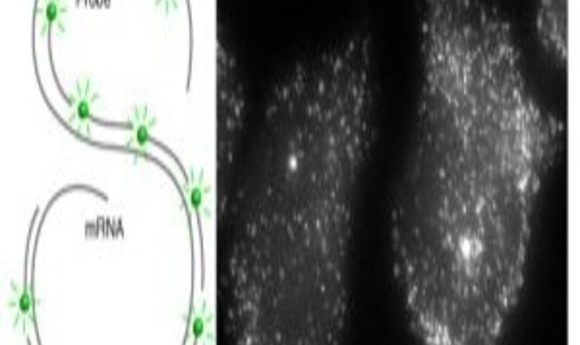
A new protocol combines single-molecule fluorescence in situ hybridization with flow cytometry to allow simultaneous, high-throughput detection of mRNA and proteins in single cells.
Precisely quantifying RNA in single cells is critical for understanding how gene expression varies within tissues or tumors. Single-molecule fluorescence in situ hybridization (sm-FISH) has proven itself an invaluable tool for studying RNA localization at the cellular level since it was first developed by Robert Singer and colleagues nearly two decades ago (1).
In contrast to RT-PCR, which measures average RNA transcript levels for a population of cells, sm-FISH targets individual transcripts with many short (~20 bases) oligonucleotide probes. Researchers conjugate each oligonucleotide to a single fluorophore that is faintly visible by microscopy.
Researchers improved the reliability of this technique by tiling ~50 oligonucleotide probes onto the same RNA, which renders each mRNA molecule so intensely fluorescent that it becomes visible as a fine spot (2). More recently, this technique has been combined with immunofluorescence to observe and quantify both proteins and RNA in individual cells. While powerful, this approach requires analyzing large numbers of single cells under a microscope, which is very time and labor-intensive.
Sanjay Tyagi and his co-workers at Rutgers University have addressed this challenge with a protocol for high-throughput sm-FISH and immunofluorescence in flow cytometry settings. Their new procedure, reported in Nature Protocols (3), can detect as few as 10 mRNA molecules per cell, as well as various cell-surface and intracellular proteins; the protocol works for both cells cultured in suspension and adherent cells.
“Although [sm-FISH] has the sensitivity of a single molecule…one has to image a large number of cells to get a sense of average behavior of a cell population. Adapting the single-molecule FISH to flow cytometry eliminates all the hard work that comes with high magnification imaging, because now thousands of cells can be imaged at once,” Tyagi said.
Tyagi’s group first tested FISH-Flow using c-fos and GAPDH (a positive control) probes in HeLa cells and observed fluorescence using microscopy and flow cytometry. They also confirmed interferon-gamma expression in CD3-positive and -negative activated T-cells and interferon-inducible GTPase (IRGA6) gene expression and protein turnover in mouse macrophages. Finally, they compared interferon gamma expression using an RNA probe in cell populations stained for different surface markers.
There are some limitations to FISH-Flow; for example, it won’t work for RNAs shorter than 500 nucleotides because it requires at least 30 probes 17–20 nucleotides in length per RNA. In addition, “Many genes are expressed at exceedingly low levels, just a few copies per cell,” Tyagi said. “We anticipate that it would be challenging to detect such sparsely expressed RNAs by FISH-Flow. New background-free in situ signal amplification techniques will have to be invented to enable their detection.”
Despite this, FISH-flow should work for most other RNAs for any gene and fluorescent probe–antibody combination as long as good positive and negative controls are included, the cells are healthy, and cells aren’t fixed for too long.