Application of bacteriophages to overcome antimicrobial resistance
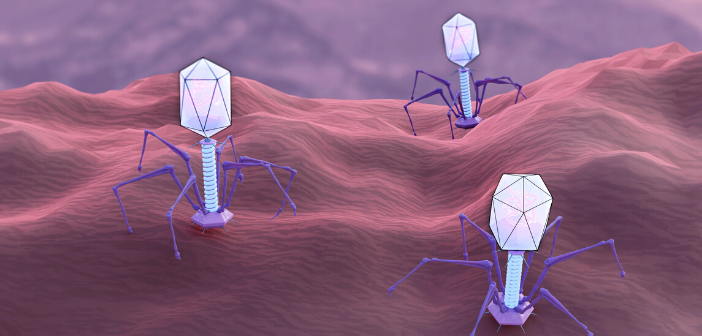
Bacteriophages (viruses that invade bacteria and can kill them) have gained renewed interest as part of measures to combat the growing tide of antimicrobial resistance. This well-documented phenomenon refers to the ability of microorganisms to resist standard antimicrobial treatments, especially antibiotics (of natural and semi-synthetic origin such as the penicillins, and purely synthetic compounds like fluoroquinolones).
The threat is enhanced where multi-drug resistance develops. Resistance emerges due to a single factor or from an accumulation of factors, including excessive and inappropriate use of antimicrobial medicines on humans and animals to poor hygiene or infection control practices. Organisms develop resistance due to adaptive responses (harboring resistance genes); through selective pressures; intrinsic resistance; or acquired resistance (via genetic transfer mechanisms: conjugation, transformation, and transduction).
Bacteriophages
A phage or bacteriophage is a virus that uses bacteria as its host. They are the most abundant organisms found throughout the Earth’s biosphere, although there are elements of biogeography, with many phages endemic to specific environments. Phages are formed of genetic material in the form of either DNA or RNA, encapsulated by a protein coat (a polyhedral). In many cases, phages kill the host bacterial cell. The types of bacteriophages of most interest as vehicles for bacterial destruction are lytic phages. These viruses disrupt the bacterial metabolism and cause the bacterium to lyse. One reason why there is an undercurrent of interest in bacteriophages is while they kill or inactivate bacterial cells, phages appear to be innocuous to human cells.
Long and erratic history of bacteriophage research
Bacteriophages were discovered back in 1915 by William Twort. A couple of years later in 1917, Felix d’Herelle noted how some phages displayed the ability to kill bacteria. In some parts of the world, notably in the Soviet Union, research into the bacterial killing properties of bacteriophages proceeded, albeit with varying levels of success. An early study, an example, demonstrated how Staphylococcus aureus phages could be deployed to treat patients suffering from purulent diseases of the lungs and pleura; and against Pseudomonas aeruginosa eye infections. Following the expansion of antibiotics, this form of antimicrobial therapy was mostly disregarded as a significant area of research, with only a handful of phages being studied in great detail.
The last decade, marked by the growing problem of antimicrobial resistance, has witnessed a renewed interest in phage biology; especially the process by which phages control many aspects of bacterial biology, and the potential for bacteriophages to be used as an antimicrobial therapy. This interest is due to four properties of the bacteriophage:
- Phages are highly specific.
- Phages are effective in lysing targeted pathogenic bacteria.
- The use of phages is safe for human hosts.
- Phages are modifiable to meet newly arising bacterial threats.
Hence, bacteriophages can serve as effective therapeutic agents in selected clinical settings.
Bacteriophages as vessels of resistance
While bacteriophages can act as killers of bacterial cells, infection with a bacteriophage can also act as a mechanism for spreading antimicrobial resistance. By the mechanism of transduction, antibiotic resistance genes can become incorporated into a phage capsule, which is later injected into another bacterium. In the process of transduction, bacterial DNA is transferred from one bacterium to another inside a virus that infects bacteria. When a phage infects a bacterium, it essentially takes over the genetic process of the bacteria to produce more phages.
During this process, bacterial DNA may inadvertently be incorporated into the new phage DNA. Upon bacterial death and lyses or breaking apart, this new phage goes on to infect other bacteria in the environment. This brings along genes from previously infected bacterium into the recipient bacterium. Sometimes this involves advantageous genes such as antibiotic resistance genes, which will leave the recipient bacterium resistant to a particular antimicrobial agent or antibiotic.
New research for new times
Most of the successes with using bacteriophages to treat infections have been with the topical treatment of skin bacterial infections. Here bacteriophages have been used as treatments of localized infections in wounds, burns, and trophic ulcers. More recently, there have been some successes reported in the form of nasal (for respiratory infections) and oral (for gastrointestinal infections) administrations. Some microbiologists are of the opinion that bacteriophages may have a role to play in personalized medical treatments.
As an example of emerging research, a study conducted at Monash University (VIC, Australia) demonstrates how effectively bacteriophages can attack and kill the bacterium Salmonella typhi, the causative agent of typhoid. This study involved the use of a purified phage solution. Essential to this was in understanding the structural mechanism by which the bacteriophage of interest attaches to the bacterial cell. This was by engaging flagella in order to reach the bacterial surface and undertake predation. A different study has shown how phages can be used to combat biofilm bacteria and thus assist with patient recovery where an infection has resulted from prosthetic-joint surgery. Key to this is with compiling a collection of phages against the particular patient’s species of bacteria to determine which combination of phages might work best.
A quite different application is with fecal virome transplantation, as an alternative to a fecal microbiota transplant. The advantage of using bacteriophages is to avoid any complications from a secondary infection. An altogether more complex approach is ‘controlled phage therapy’, albeit one that remains as yet at the cell culture stage. Here pages are conjugated gold nanorods and applied to bacteria. When exposed to near-infrared light (photo-excitation) the energy is converted from light to heat. The heat is sufficient to kill the bacteria and also the phages, providing a safeguard against any subsequent evolutions.
In a final example, researchers from McMaster University (ON, Canada) are experimenting with formulating an antibacterial gel containing 300 trillion phages, as a topical application (as structured hydrogels of self-organized M13 bacteriophage bundles).
There remain obstacles towards developing large-scale and commercial phage therapy. For instance, purifying and growing phages can be a long, complex and challenging process. One area of concern is with the human body’s reticuloendothelial system, which functions to clear phages from the body. This serves to lower the number of phages, which could lead to a level of phages too low to effectively defeat infecting bacteria. The body can also, on occasion, develop phage-neutralizing antibodies with a similar result. Furthermore, some microbiologists are of the view that eventually bacteria will evolve to develop resistance to specific types of phages.
Turning bacteriophage research into the development of widespread and effective therapies remains at an early stage. Nonetheless, the successes reported so far demonstrate that the use of bacteriophages represents a promising area of research to develop an effective treatment with which to help stem the flow of antimicrobial resistant bacteria.