Combining engineering with medicine: an interview with Brenda Wilson
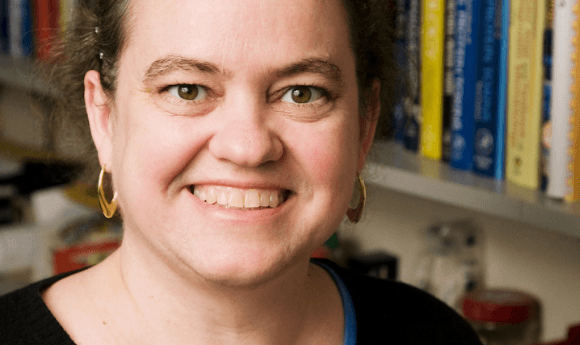
Brenda Wilson is a professor of microbiology who is currently undertaking novel multidisciplinary research on human and primate microbiomes, as well as developing novel anti-toxin therapeutics and toxin-based therapeutic delivery platforms.
We caught up with her at the recent ASM Microbe (San Francisco, CA, USA, 20–25 June 2019) to chat about her research into and new ways to combine engineering and medicine.
Can you introduce yourself and give us a brief overview of your research and education focuses?
I am a professor in the Department of Microbiology at the University of Illinois at Urbana-Champaign (IL, USA). I am also affiliated with the Institute for Genomic Biology (IL, USA), where I am part of a team looking at mining microbial genomes for various therapeutic purposes. I am also an inaugural professor at the new Carle Illinois College of Medicine (IL, USA), which is engineering focused and where we have a new mindset of integrating engineering with medicine. We hope to get the students that graduate with us into a different type of thinking, using more problem-solving approaches, with a focus on driving innovation.
New technologies are coming along that allow for all kinds of diagnostics and monitoring, these kinds of technologies can then be integrated with the biological aspects of infectious diseases, of pathology or of cancer, really of anything that is involved in medicine. When you think of engineering you think of devices. Devices are a powerful tool that we can use as a multifactorial way of approaching medicine, but we also want students to see medicine in a very holistic way. The idea is that we would be training students in thinking about things from an engineering point of view and trying to figure out how to integrate a lot of different factors and technologies into a solution for complex medical problems.
Going over to the gut microbiome, what can the gut microbiome tell us about our health?
I first came into this field of research before it was called the microbiome. We originally started in the Institute for Genomic Biology as a group that was studying host microbe systems. We felt that one of the areas that had not been approached very well was these polymicrobial infections — or shifts in microbiota— that would happen inside your gut or vaginal system and how that would then impact your health, as well as disease.
At that time everybody was thinking more in terms of disease, but we felt that what we really want to know is, how can we promote the health of the microbial communities within our bodies? We originally focused on the vaginal system. Hardly anybody was working in that area and very little was known other than just a couple of key dominant microbes that were involved in human health, but there were no really ideal animals to work with that truly modelled the human system. Therefore, we ended up initially investigating nonhuman primates. As a result of that, we worked with anthropologists to look at the coevolution of our microbiomes as we developed as a species. One of our key discoveries was that humans are the only ones that have Lactobacillus as the dominant microbe in their vaginal system. We are still trying to figure out why that is the case.
Then we spread out to the gut as well, looking at how nutrition impacts our microbiomes and health. We learned that what constitutes healthy is actually very broad and can be specific to the individual. Our microbiomes are unique to us and of course, if that’s the case, then how is it that this uniqueness affects us when we get sick? How does what we eat impact our cognitive function and our development from infant on up, or even as a fetus? How does that impact our health, short-term and long-term? And how do changes in our gut microbiomes result in either a predilection towards disease or caused the disease itself?
We now know that changes in the microbiota will affect the host, and the interplay between the host and its microbiota makes all the difference. The sequence of events such as illness or dietary changes that you have previously been exposed to will indeed cause a difference in the body’s response the next time you are exposed. We can sometimes predict when there might be an adverse outcome, but not always, and so we are still learning about the factors that trigger adverse outcomes. For example, we initially thought in Clostridium difficile infections that fecal microbiota transplants would be the solution, and we were all going for it. We identified certain individuals who were good donors and started scaling up the use of this approach for treating or preventing a lot of different diseases involving gut microbiota, but we got a surprise recently when two people got very, very ill from having received fecal microbiota transplants, and one person died. Hundreds of other people who have already gone through this procedure did not have such an adverse outcome. We still don’t fully understand what happened and are continuing to investigate, but we are now moving forward with much more caution.
How do you hope to make progress with increasing the understanding of the microbiota?
With an increase in understanding in metagenomics, studies are becoming a lot more reliable and thorough. We can now find out what the metabolic potentials of microbial communities are and can investigate them to determine which particular product of a microbial genome is contributing to the phenotype that we are seeing. For example, one of the things that we have been finding is that some microbes make protein toxins. A number of genes in the genomes of bacteria found in our microbiomes appear to encode proteins have modules that are very similar to these protein toxins. We can find these sequences within a large number bacterial genomes and genome assemblies that we have currently available in our databases.
For example, in the last few years, we have discovered that yes, if you have a specific toxin-encoding gene called cnf, you have a much higher risk of getting cancer. Some individuals who have a particular genotype of Escherichia coli in their gut microbiota also have a much higher rate of colorectal cancer and prostate cancer.
Some people never get cancer, they are not going to get it and others seem to have a predilection; why? How does that happen? I think that we are now coming into an era where we are going to start being able to address these kinds of questions by also considering what kinds of microbes we are exposed.
-
Exploring the future of fecal microbiota transplantations
-
Finding therapies for shigella infections
-
Expanding the hygiene hypothesis
How are genomic technologies being utilized to study these interactions between the host and the microbes?
One of the interesting things about this is, before we were just using short sequence reads of 16S rRNA genes to find out what types of microbes were present. Now, we can get much longer sequence reads and, as a result, we can pull out entire metabolic pathways and find genes that are involved in various biosynthetic pathways, involved in making various molecules or metabolites. Consequently, we now are able to get a good understanding of the metabolic potential that’s there. We can then follow that up with how many of those genes are being expressed? How many of them make proteins? How many types of metabolites are being made by the microbes? We can do all that using transcriptomics and metabolomics.
In addition to merging genomics with transcriptomics, we are now going a step further with proteomics and metabolomics. Whole linkages now can be correlated with each other and we can do that not only in the bacterium, which we were kind of confined to before, but we can also do it simultaneously in the host. We can see when the expression levels of certain genes are coordinated with each or when one goes up, and the other one goes down. You can now look at those differences and the dynamics that are going on in almost real time and start to build predictive models of what will happen. It’s not quite there yet, but I see, in the near future, that it’s going to be possible to anticipate onset or escalation of certain types of diseases.
For example, some microbes expressing certain products influence and interact with the host and the host’s response to this can now be seen and dealt with through interventions, if needed. Some pathogens take advantage of these host responses, which allows them to utilize the hosts, promoting colonization and propagation in order to gain nutrients and establish a colony. The host is just another environment that they are adapting to, and some microbes are better at it than others. I think these new technologies are going to allow us to generate better models of health and disease that we can test and use for screening new treatments.
What challenges do you face with this technology and how do you hope to overcome them?
One of the challenges of this technology is we are not seeing these patterns in high resolution. We are trying to develop some new algorithms and bioinformatic approaches that will allow us to handle some of the artifacts associated with the sequencing technology and help to mitigate them. However, to address some of those challenges, we must address the chimera issue; anytime you have a sequencing technology that generates chimeric sequences, such as PCR, that tends to generate different amounts of chimeras, depending upon your sample, you will have very different percentages of chimeric reads among different samples. This in turn will impact the results interpretation of the data.
We are discovering that, when you do have a method of handling these chimeras and you include them in your calculations, it does change things. It changes the profile, in terms of the composition, and it changes the outcome and the diversity. We just recently came up with a bioinformatics tool called ‘BlastBin’ that can be used to handle chimeric sequence reads, which we presented as a poster here at ASM Microbe. The idea is that we could put the algorithm tool into the existing bioinformatics pipelines, allowing you to count the chimeras and calculate how much each contributing bacterial DNA template was present in the sample, and then utilize this data in the final analysis.
In practice, we discovered that the samples with chimera sequence reads matched closer to the metagenomic data that we had from the same sample. It’s not identical, because, of course, there’s always issues with measuring precisely metagenomic data as well, but at least it was a closer match.
Could you explain how your research could impact the development of toxin-based therapeutics?
In the process of learning about toxins, we now have a greater understanding of what cellular substrates they act on, what receptors and host cells they target and how they are able to bind to a host cell and deliver their toxic cargo to the interior cytosol of that cell, so as to gain access to their substrates and cause toxic effects that lead to disease.
One of the key questions surrounding novel therapeutics that are based on antibodies and enzymes, called biologics, is how can you get a therapeutic cargo like that delivered across the cell membrane and into the cytosol? Well, it turns out the toxins can do this naturally. Therefore, we developed a cargo-delivery platform called bacterial toxin-inspired drug delivery (BTIDD), which allows us to swap out the toxic part with a therapeutic cargo. We can also swap out the toxin’s receptor binding domain with other proteins that can bind to specific recognition sequences for whatever host target cell we want.
We now have the basis of a drug delivery system for biologics that will travel specifically to a targeted host cell, get across the membrane of that cell and deliver the biologics specifically into the cytosol of the target cell. Our first prototype was trying to prevent or reverse the paralysis associated by botulism food poisoning. To do this, we used the botulinum neurotoxin itself as it is highly specific at targeting nerve cells and causing botulism paralysis. In a clinical scenario you can give a paralyzed patient antitoxin antibodies to block any further intoxication, but how are you going to stop it from continuing to cause paralysis once the toxin has entered the nerve cell? Botulinum neurotoxin contains a protease domain that cleaves the proteins inside the nerve cells, preventing neural transmission. By swapping out that protease domain of the toxin with a specific antibody that targets the toxin’s protease domain or with a substrate inhibitor that blocks the activity of the protease domain, we can get the antibody or inhibitor delivered to the nerve cells, where it can prevent this continuous paralysis.
Is there anything else that you would like to add?
Right now, everything is so costly, it’s really challenging for individual labs be able to support themselves. In fact, most of the money usually just goes towards supporting people more than anything else. I don’t think the general public realizes the whole potential of where science is going and how science works. I try to do a lot of outreach and public education surrounding scientific literacy, so that they understand the importance of what scientists are doing.
I think that it would be really beneficial for us to have more outreach of this nature, to let people know about how scientists work and why we can’t immediately always have a result the next day. It does take a long build-up of findings, like the BTIDD system I just described, which took us over 10 years to develop. People are seeing and hearing about the microbiome, but they don’t necessarily understand it. I am encouraged that they do know about it, and I am hoping that more folks learning about its importance. For instance, we now know that the microbiome can impact cognition, infant and child development and even the development of our immune systems. In more recent years, it has been discovered that the therapeutic impact of PD-1 inhibitors can be correlated with and affected by the composition of an individual’s microbiome. Therefore, we are moving closer towards tailoring medicine towards the individual.