Dan Portnoy on Listeria monocytogenes
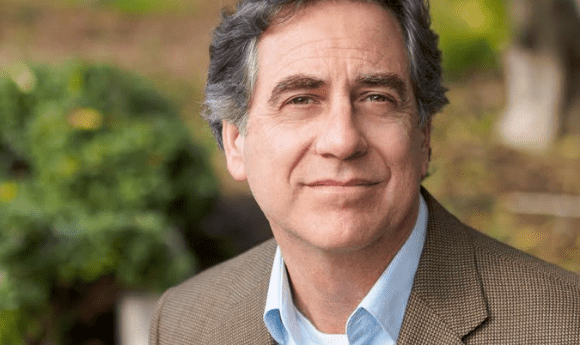
At ASM Microbe 2019, we caught up with Dan Portnoy (University of California, Berkeley; CA, USA) to talk about his research into the bacteria Listeria monocytogenes. Dan discussed the bacteria’s applications as a model for host-pathogen interactions and its potential as a vaccine for both infectious diseases and cancer.
Please can you introduce yourself and provide a brief overview of your research focuses?
I am a professor at the University of California, Berkeley (CA, USA), and as a microbiologist, my main research focus is in infectious disease, but more specifically, intracellular pathogens. I work on a facultative intracellular bacterial pathogen called Listeria moncytogenes, a food-borne pathogen, which has a great mouse model for infection that has been used for over 50 years as a way to understand basic aspects of immunology. The major observations have shown that when you administer Listeria to a mouse at a sub-lethal dose, the mouse will counteract and clear the infection within a week, after which it’s immune to subsequent challenge.
While most vaccines target production of antibodies, the type of immunity that is required to protect against Listeria is called cell-mediated immunity, where cytotoxic T-cells recognize infected cells and kill them. In the case of Listeria, it is highly effective, leading me, and others, to consider Listeria as a potential vaccine vector. Another important classic observation is that you cannot immunize mice with killed Listeria; it has to be live, replicating bacteria. That’s a pretty fundamental observation that we have been trying to understand for years.
Could you outline your research on the virulence factor LLO?
A lot of the work in my lab involves attempting to understand the basic biology of Listeria. We learned early on that bacteria cannot grow when initially internalized into a membrane-bound compartment (a phagosome), and so the pathogen needs to produce a pore-forming molecule called listeriolysin O (LLO). LLO inserts into the phagosome and makes a large pore that results in the lysis of the phagosome. Bacteria then enter into the cytosol where they grow rapidly and release antigens, which are then recognized by the immune system. They also induce various cytokines or induce the macrophage or other cells to produce various cytokines.
Please can you tell us about your research on vaccine development for both infectious diseases and cancer?
The idea that Listeria could be used as a vaccine to induce cell-mediated immunity to a foreign antigen arose largely because of the cell biology of infection. The bacteria are found in the cytosol of the host cell where antigens are processed and presented to the immune system, leading to the generation of antigen-specific CD8 T cells that can recognize infected cells and kill them.
Originally, I thought that this could be a vaccine for other intracellular pathogens including viruses and protozoa. I was thinking about major diseases of our time such as HIV, malaria, and tuberculosis – that is where my interests were. It turns out that immunity to cancer and immunity to intracellular pathogens have some features in common – in both cases you have a cell that has to be recognized by the immune system. The idea that Listeria could be used for a therapeutic cancer vaccine started in the mid-90s when Yvonne Paterson (University of Pennsylvania; PA, USA) collaborated with Drew Pardoll (Johns Hopkins University; MD, USA). Using a strain of Listeria, which we had already constructed that expressed an influenza protein, they showed that Listeria could be used as an immunotherapeutic to treat mice with a tumor expressing the influenza antigen. That was the first paper published showing that Listeria had potential as a cancer vaccine.
How has the field evolved since that initial paper?
Prior to my move to the University of California, Berkeley, I was at the University of Pennsylvania, and although I worked with Yvonne Patterson, I decided at that time not to work on vaccines. Yvonne continued producing papers in that field and started a company called Advaxis (NJ, USA). Around the same time, after I moved to Berkeley, where I have now been for 22 years, a local biotech company named Cerus initiated a cancer immunotherapy program. They contacted me and I ended up working with them as a consultant, a collaborator, and a science advisory board member.
Over the years, that company morphed, eventually forming a spin-off company. This company was then picked up by Aduro Biotech (CA, USA), and I worked with them for about 15 years, researching how to develop Listeria vaccines for cancer. Both Aduro and Advaxis began conducting clinical trials in parallel. Initially, we decided on the ActA-minus strain for use in trials, first in mice but then eventually in humans. For a Listeria vaccine to work, bacteria need to be able to escape the phagosome and then enter the cytosol. If they can’t get out of the vacuole, then they can’t grow and they don’t immunize. Once bacteria get into the cytosol, they produce a protein called ActA, the essential virulence factor that allows the bacteria to spread from cell to cell. If you mutate that ActA protein, the bacteria still enter the cytosol and they still grow, but they’re attenuated by over 1000-fold.
This mechanism became the primary basis of attenuation that Aduro used to ensure that the very potent vaccine would be safe. Since the ActA protein is so well expressed, the basis of the vaccine was to create a fusion protein consisting of a tumor antigen fused to ActA, and then get Listeria to secrete this protein. Upon phagocytosis, Listeria enters a cell’s cytosol and secretes that tumor antigen, which now is processed and presented. In a mouse model, this produced a great CD8 T-cell response that protects the mice in a therapeutic cancer model. Aduro started a series of small trials using Listeria expressing an antigen called mesothelin, a human protein that is overly represented on various different cancers. Some of the significant clinical trials at Aduro were targeting pancreatic cancer, whereas the trials carried out by Advaxis were targeting other types of cancers. Over the years, both groups had a mix of promising and disappointing results, but it still has a lot of potential.
Why is Listeria monocytogenes such a good model system for understanding host–pathogen interactions?
Many infectious disease agents that are considered important, due to how many people get ill or die from them, are actually very difficult to work on. They’re either slow growing, don’t have a good animal model, or are very hard to manipulate. Listeria avoids all those challenges because they grow rapidly, are amenable to genetic manipulation and have a great mouse model. Also, very importantly, they don’t kill infected host cells. However, when we isolate mutants or construct Listeria strains that do induce cell death, it becomes avirulent. That ability to grow without killing cells is critical for their pathogenesis, which makes some sense when it’s an intracellular pathogen. When they kill the cell, they lose their home, and that’s the end of it. Listeria has a number of mechanisms to prevent cell death.
Another interesting feature is that its pathogenesis might not be as complicated as other pathogens. For example, all pathogens secrete proteins that mediate their pathogenesis, allowing them to cause disease or to grow in cells. Some secrete dozens if not hundreds of effectors and knocking out one or another often doesn’t have a very striking phenotype. But with Listeria, there are relatively few virulence factors and in many cases the mutations that we make have very striking phenotypes. For example, if you knock out LLO, the bacteria can’t get out of the phagosome, so they don’t grow and are 100,000-fold less virulent. If you knock out the ActA gene, the bacteria will break out of the phagosome but they can’t spread cell to cell and so they’re 1000-fold less virulent. These are both striking phenotypes. We can study this in vitro in the lab using microscopy, mouse models, and other methods.
There are two major features of all Listeria that suggest it may be a good vaccine. The first is that they can get to the cytosol and don’t kill the host cell. The second is the ability to induce the proper cytokines that the host cells make to stimulate the immune system. We asked how Listeria triggers the host’s immune system. We started out by looking at genes that were induced by wild-type Listeria but not by the strain that lacked LLO. We found that there was one small set of host genes typified by type 1 interferon that the wild-type strains induce but that the mutants lacking LLO do not. We knew from the literature that this was a pathway that would involve a transcription factor called IRF3, and we saw Listeria induce all these genes that were activated by IRF3.
How can bacterial metabolism impact host innate immunity?
I collaborated with a number of other Bay Area investigators studying intracellular pathogens, such as Jeff Cox (University of California, Berkeley), who was working with Mycobacterium tuberculosis at University of California, San Francisco (CA, USA) at the time, and Russell Vance, here at Berkeley, who was working on Legionella pneumophila. We noticed that all of these live, intracellular pathogens induced the pathway that produced interferon. We took a genetic approach, screening 10,000 transposon mutants of Listeria, and looked for mutants that induced more or less interferon. This strategy led to a series of studies in which we discovered a mutant that secreted more interferon and also overexpressed a multi-drug efflux pump. We hypothesized that Listeria was secreting something through these multidrug efflux pumps, and Josh Woodward (University of Washington; WA, USA), a postdoc in my lab at the time, used biochemical approaches to find that Listeria was secreting a molecule called cyclic-di-AMP (c-di-AMP). This represented the discovery of a new molecule since there was only one report of c-di-AMP, although there were reports of cyclic-di-GMP (c-di-GMP), which also induces interferon.
Since then, it’s been found that dozens of other bacteria make c-di-AMP as well. It turns out that c-di-AMP in Listeria, and many other bacteria, is not only secreted to activate the immune system but is also an essential molecule for the bacteria themselves for controlling aspects of bacterial metabolism. While we were doing these studies, Vance was performing ENU mutagenesis in mice and isolating the macrophages. As a collaboration between our labs, we screened for macrophages that would not produce an interferon response to Listeria. One mutant, named the Goldenticket mouse, did not express interferon in response to Listeria due to a mutation in a gene called STING (stimulator of interferon genes) that had previously been found to be involved in the response to viruses. From the literature, we knew that DNA activated STING though it didn’t bind directly, but Vance discovered that c-di-AMP and c-di-GMP both bound to and activated STING. We now know that this response of turning on cytokines with Listeria was dependent on the production of c-di-AMP that binds to STING, which then leads to activation of IRF3 and turns on these genes. Other studies have shown that many bacteria activate STING the same way as Listeria, but more often it turns out that the interferon response is activated by the release of microbial DNA.
James Chen (University of Texas Southwestern Medical Centre; TX, USA) discovered that humans and mice have a protein called cyclic GMP-AMP synthase (cGAS). When cGAS binds DNA, it synthesizes another cyclic-di-nucleotide, c-di-GAMP (cGAMP), which binds and activates STING. We now understand that almost all intracellular pathogens can activate STING either by directly secreting their own cyclic-di-nucleotide or by activating cGAS that then leads to immune system activation. Aduro has examined whether cyclic-di-nucleotides themselves could be anti-cancer, and it turns out that they can inject modified derivatives of c-di-AMP directly into tumors in mice and then all tumors are eradicated. That worked amazingly well, and these mice are now resistant to subsequent re-administration of the tumor. Now, Aduro has partnered up with Novartis, and they are currently conducting human clinical trials using cyclic-di-nucleotides as anti-cancer therapies. There are other companies doing this as well, and though this is not something I personally work on in my laboratory, it is gratifying that these developments are derived from our research.
What tips do you have for early -career researchers in your field?
As a microbiologist, I believe that a lot of answers can be found simply by studying the basic biology of microbes. Studying how Listeria grows in cells and causes disease led to uncovering some very basic information that may have clinical relevance. For example, c-di-AMP is really just a secondary messenger that the bacteria use for their own metabolism, and it was surprising that it had not been discovered previously. Just by asking how the host recognizes bacterium, we discovered a new essential bacterial molecule. By understanding how the host sees the bug and how the bug avoids being seen, you can learn so many basic aspects of biology, many of which have potential clinical relevance.
The key thing that I would advise is not to go in with preconceived ideas. Have an open mind and devise experiments that will open other areas. You never know what you’re going to find! We had no idea bacterial multidrug efflux pumps or cyclic-di-nucleotides were involved in this response, but we just looked for mutants and that’s what we found. Unbiased approaches to figuring out complex processes can be very successful.
Catch up on the rest of our coverage of ASM Microbe 2019 in our Spotlight feature