How do prokaryotes expel calcium?
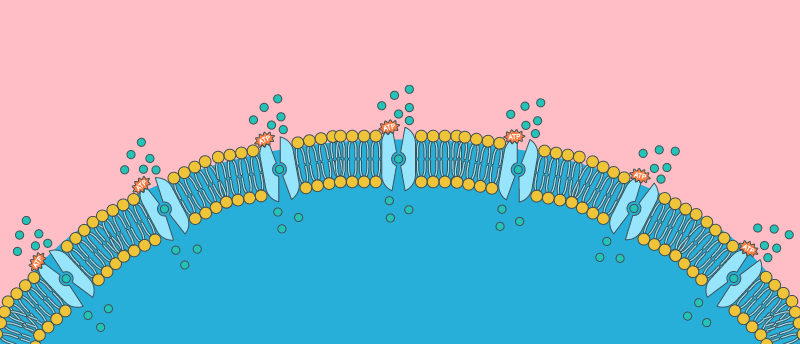
A new method exposes key details of the mechanism behind calcium signaling in prokaryotes for the first time.
Working in a Listeria monocytogenes model, researchers from Umeå University (Sweden) led by Magnus Andersson have elucidated key aspects of the previously mysterious procaryotic mechanisms for calcium signaling. The team used a combination of methods, including a specially developed technique involving the use of synchrotron X-rays, and their findings highlight a potential target for future antimicrobial therapeutics.
Calcium signaling plays a vital role in both eukaryotic and prokaryotic cellular processes, but the mechanisms behind calcium transport are more thoroughly understood in the former. Burgeoning research into prokaryotic calcium signaling has so far revealed an L. monocytogenes membrane protein – Ca2+-ATPase 1 (LMCA1) – as a key player in the efflux of calcium from bacterial cells during signaling responses.
This protein has been shown to play a key role in the survival of L. monocytogenes within phagosomes and in alkaline environments, helping to maintain internal calcium concentrations and therefore protecting the function of bacterial cells. The ability to survive in alkaline environments makes L. monocytogenes a frequent culprit in food poisoning cases, as the bacteria can survive the mild alkaline treatments utilized in commercial food processing.
Cocktail of viruses holds promise as an alternative treatment for Klebsiella pneumoniae infections
A cocktail of bacteria-fighting viruses has been concocted with the potential to successfully treat antibiotic-resistant Klebsiella pneumoniae infections.
Setting out to understand this protein and its properties, the team deployed cryo-EM to explore the differences between calcium binding sites in LMCA1 and the eukaryotic P-type ATPase PMCA. The team also developed a method using synchrotron X-rays in time-resolved X-ray solution scattering to determine the changing structure of proteins as they execute their functions, which they utilized alongside smFRET experiments to explore characteristics and functions of LMCA1 such as its overall conformation, ion pathways and binding sites.
The team identified a key difference in the mechanism of LMCA1 verses that established in its closest mammalian homolog, a protein called SERCA. In broad strokes, Ca2+-ATPases function by switching between two states: E1, where the cytosolic portion of the protein is open and intracellular calcium can enter; and E2, during which the extracellular section of the protein is open and the calcium can be released. The team identified that for LMCA1, ATP-dependent phosphorylation during E1 was the rate-limiting step during calcium transport, rather than the E1P to E2P transitions in SERCA1a.
Commenting on the impact of these findings, Andersson stated that by, “identifying clear differences between calcium transport in eukaryotic and prokaryotic systems, our study has taken an important step toward the development of new antimicrobial strategies and ensuring safety in food production.”
The results have been so well received that the team has secured funding from the NIH (MD, USA) for a new collaboration with the University of Michigan (MI, USA). This should enable the team to implement the methods they have developed in the design of molecules that “can activate calcium transport proteins in cases of heart failure,” says Andersson.