Precision medicine in sepsis: where do we begin?
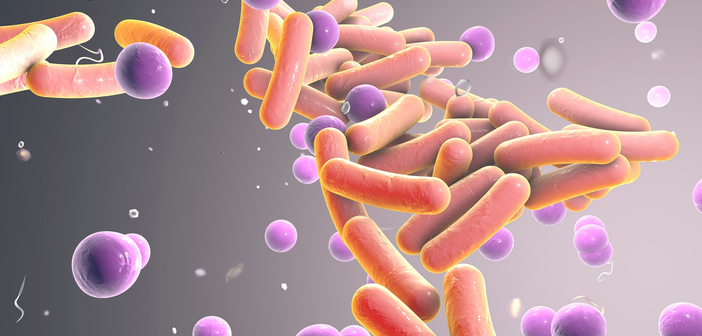
Sepsis arises from a dysregulated host immune response to infection. Global sepsis burden was estimated to be 48.9 million cases in 2017 in a recent study and mortality was found to be twice than previously estimated, accounting for 1 in 5 deaths worldwide [1]. Despite advances in critical care medicine, sepsis morbidity and mortality remain high.
In the battle against sepsis, taking a more personalized approach has become inevitable since ‘one size’ does not ‘fit all’, as shown from numerous clinical trials. The reason is that septic patients comprise of a heterogeneous population of individuals who suffer from different infections, against which their immune system does not have the same type and level of response and who do not respond to treatment similarly due to variable host factors, genetic predisposition, comorbidities, pathogen load and/or type of infection. Recently a position paper by the European Society of Clinical Microbiology and Infectious Diseases, acknowledging that precision medicine can serve as an opportunity to improve outcome in sepsis [2], has outlined a roadmap for a more personalized approach in the management of this syndrome [3].
Precision (or personalized) medicine in sepsis entails making an early and accurate microbiologic diagnosis, determining the host immune response signature and assessing individual response to treatment, in order to tailor therapy to the specific needs of each individual patient.
So how can we make sepsis management more precise and where do we begin?
In my opinion, we have to begin with securing a rapid and accurate and microbiologic diagnosis. It has been shown that appropriate and prompt initiation of antimicrobial therapy strongly affects survival [4]. Choosing the right antimicrobial may be rather straightforward in sepsis due to community-acquired infections manifesting in a typical manner. However, in primary bacteremia, nosocomial infections and infections presenting atypically, choosing wisely and getting the antibiotic right from the start can be more challenging. Moreover, in the era of antimicrobial resistance, especially in institutions where multi-drug resistant pathogens are endemic, broad-spectrum combination of antimicrobials is often recommended as an initial therapeutic approach for septic patients. However, increased antimicrobial consumption leads to more antibiotic resistance [5]. Moreover, empirical treatment frequently fails. A study by Byl and colleagues [6] showed that empirical treatment was adequate only in 63% of episodes of bacteremia. Ineffective initial empirical antibiotic regimens were independently associated with increased mortality [7–9].
“In my opinion, we have to begin with securing a rapid and accurate and microbiologic diagnosis.”
Establishing a correct microbiologic diagnosis early is paramount, primarily for the patient, but also for antimicrobial stewardship purposes [10]. It is true that conventional culture methods often fail to provide a rapid microbiologic diagnosis. Microbiological diagnosis of the causative organism in sepsis is feasible only in half of the cases and usually the full antimicrobial susceptibility report for the isolated pathogen is available on average 2–3 days after sample collection. Molecular diagnostic tests have higher sensitivity compared with conventional microbiology and could therefore assist in an earlier (within a few hours) detection of the causative organism, permit the timely initiation of appropriate antimicrobial therapy, reduce total inappropriate antibiotic exposure and decrease related length of hospital stay and healthcare costs. In addition, these novel methods may be a valuable tool for pathogen detection in antimicrobial-pretreated patients with sepsis [11–13].
Rapid diagnostic tests for bacterial detection can be used on positive cultures and directly from blood or other biologic samples [3,14]. Platforms used in positive cultures are multiplex polymerase chain reaction (PCR) assays, fluorescent in situ hybridization (FISH) and matrix-assisted laser desorption/ionization-time of flight (MALDI-TOF) mass spectrometry. More recently, methods that detect pathogens directly from blood have been developed for clinical use and include mainly multiplex polymerase chain reaction (PCR) assays alone or in combination with other technologies like magnetic resonance technology and next generation sequencing (NGS) [3,15].
Assays that are used in positive cultures have been more widely validated and have good sensitivity [3]. However, when it comes to blood cultures the limitation of having to wait for the culture to become positive decreases their impact on the timeliness of targeted therapy. Pathogen detection directly from blood is very promising in that it can yield a result within a few hours. Moreover, NGS, whose diagnostic yield extends beyond a predefined panel of specific pathogens, can detect even small amounts of bacterial nucleic acid. Needless to say, that NGS usually requires depletion of human DNA and bacterial DNA extraction as a first step before that sample can be analyzed [12,15].
“Nonetheless, rapid molecular tests when used with antimicrobial stewardship programs, are cost-effective and can even result in the reduction of healthcare expenditures…”
The pitfalls of molecular diagnostic tests can be false positive results due to the detection of bacterial nucleic acid of no or doubtful clinical importance, or false negative results due to suboptimal sample preparation. However, one can expect the latter to be minimized with technical optimization of future platforms and ‘one step’ processing. Another downside of these tests is that they do not provide a full antibiotic susceptibility profile. However, some multiplex PCR platforms have incorporated resistance genes in their detection panel and next generation sequence can provide information on the resistance and virulence potential of the detected pathogen [3]. However, these tests still have variable sensitivity and thus clinicians cannot solely rely on them yet. Nonetheless, rapid molecular tests when used with antimicrobial stewardship programs, are cost-effective and can even result in the reduction of healthcare expenditures [11].
In parallel to making an accurate microbiologic diagnosis early, an equally crucial step towards the implementation of precision medicine in sepsis is to characterize sepsis endotypes and further perform risk stratification using biomarkers derived from omics (genomics, epigenomics, transcriptomics, proeomics, metabolomics) technologies. Septic patients have both different and dynamic immune response profiles making it necessary to determine each time the biologic pathways responsible for the dysregulation of the host response (i.e., immunoparalysis or excessive inflammation). Omics-based biomarkers, once validated, can help define clinical sub-groups of patients with similar biologic characteristics as well as inform prognosis [16–18]. It is more likely that a panel of biomarkers will be more suitable than one specific biomarker to provide a snapshot of the culprit-altered biologic pathway. Furthermore, NGS technology has been used in sepsis to assess both host and pathogen profiles from one sample [19]. Biomarkers can also be used to assess individual treatment response after targeted therapy aiming to restore a specific biologic pathway [20]. Genomic and transcriptomic biomarkers have been developed, however, these technologies still have increased cost and turn-around times that so far preclude them from use in everyday practice [16,21,22]. Nevertheless, designing interventions, which are specific to subgroups sharing a common pathophysiologic profile, would have a better chance of achieving a favorable outcome in sepsis and relevant biomarkers should be validated in clinical trials.
Precision medicine in sepsis has taken its first steps but we are still quite far from a comprehensive implementation. In cancer, precision therapies target a specific molecular signature, leading to improved outcomes. In sepsis though, endotypes depend on both host and pathogen factors as well as their unique interplay. Hence, in order to design a targeted therapeutic approach, it will be necessary to optimize the timeliness and accuracy of diagnostic methods and to analyze complex and dynamic data using advanced computational methods.
- Rudd KE, Johnson SC, Agesa KM et al. Global, regional, and national sepsis incidence and mortality, 1990–2017: analysis for the Global Burden of Disease Study. Lancet 395(10219), 200–211 (2020).
- Van Der Poll T, Van De Veerdonk FL, Scicluna BP, Netea MG. The immunopathology of sepsis and potential therapeutic targets. Nat. Rev. Immunol. 17(7), 407–420 (2017).
- Rello J, van Engelen TSR, Alp E et al. Towards precision medicine in sepsis: a position paper from the European Society of Clinical Microbiology and Infectious Diseases. Clinical Microbiology and Infection. Clin. Microbiol. Infect. 24(12), 1264–1272 (2018).
- Rhodes A, Evans LE, Alhazzani W et al. Surviving Sepsis Campaign. Crit. Care Med. 45(3), 486–552 (2017).
- Versporten A, Zarb P, Caniaux I et al. Antimicrobial consumption and resistance in adult hospital inpatients in 53 countries: results of an internet-based global point prevalence survey. Lancet Glob. Heal. 6(6), E619–E629 (2018).
- Byl B, Clevenbergh P, Jacobs F et al. Impact of infectious diseases specialists and microbiological data on the appropriateness of antimicrobial therapy for bacteremia. Clin. Infect. Dis. 29(1), 60–6 (1999).
- Ibrahim EH, Sherman G, Ward S, Fraser VJ, Kollef MH. The influence of inadequate antimicrobial treatment of bloodstream infections on patient outcomes in the ICU setting. Chest 118(1), 146–155 (2000).
- Garnacho-Montero J, Gutiérrez-Pizarraya A, Escoresca-Ortega A, Fernández-Delgado E, López-Sánchez JM. Adequate antibiotic therapy prior to ICU admission in patients with severe sepsis and septic shock reduces hospital mortality. Crit. Care. 19(1), 302 (2015).
- Tacconelli E, Cataldo MA, Mutters NT et al. Role of place of acquisition and inappropriate empirical antibiotic therapy on the outcome of extended-spectrum β-lactamase-producing Enterobacteriaceae infections. Int. J. Antimicrob. Agents. 54(1), 49–54 (2019).
- Bassetti M, Poulakou G, Ruppe E, Bouza E, Van Hal SJ, Brink A. Antimicrobial resistance in the next 30 years, humankind, bugs and drugs: a visionary approach. Intensive Care Med. 43(10), 1464–1475 (2017).
- Pliakos EE, Andreatos N, Shehadeh F, Ziakas PD, Mylonakis E. The cost-effectiveness of rapid diagnostic testing for the diagnosis of bloodstream infections with or without antimicrobial stewardship. Clin. Microbiol. Rev. 31(3), e00095–17 (2018).
- Mangioni D, Viaggi B, Giani T et al. Diagnostic stewardship for sepsis: The need for risk stratification to triage patients for fast microbiology workflows. Future Microbiol. 14(3) 169–174 (2019).
- Buehler SS, Madison B, Snyder SR et al. Effectiveness of practices to increase timeliness of providing targeted therapy for inpatients with bloodstream infections: A laboratory medicine best practices systematic review and meta-analysis. Clin. Microbiol. Rev. 29(1), 59–103 (2015).
- Dubourg G, Raoult D. Emerging methodologies for pathogen identification in positive blood culture testing. Expert Rev. Mol. Diagn. 16(1) 97–111 (2016).
- Mangioni D, Peri AM, Rossolini GM et al. Towards rapid sepsis diagnosis and patients stratification: what’s new from microbiology and omics science. J. Infect. Dis. doi:10.1093/infdis/jiz585 (2019).
- McHugh L, Seldon TA, Brandon RA et al. A molecular host response assay to discriminate between sepsis and infection-negative systemic inflammation in critically ill patients: discovery and validation in independent cohorts. PLoS Med. 12(12), e1001916 (2015).
- Davenport EE, Burnham KL, Radhakrishnan J et al. Genomic landscape of the individual host response and outcomes in sepsis: A prospective cohort study. Lancet Respir. Med. 4(4), 259–271 (2016).
- Scicluna BP, van Vught LA, Zwinderman AH et al. Classification of patients with sepsis according to blood genomic endotype: a prospective cohort study. Lancet Respir. Med. 5(10), 816–826 (2017).
- Langelier C, Kalantar KK, Chhristenson S et al. Precision diagnosis of lower respiratory tract infections in the ICU via metagenomic next generation sequencing. presetned at Am. J. Respir. Crit. Care Med. 197, A4459 (2018).
- Wong HR, Cvijanovich NZ, Anas N et al. Endotype transitions during the acute phase of pediatric septic shock reflect changing risk and treatment response. Crit. Care Med. 46(3), e242–9 (2018).
- Tsalik EL, Henao R, Nichols M et al. Host gene expression classifiers diagnose acute respiratory illness etiology. Sci. Transl. Med. 8(322), 322ra11 (2016).
- Scicluna BP, Klein Klouwenberg PMC et al. A molecular biomarker to diagnose community-acquired pneumonia on intensive care unit admission. Am. J. Respir. Crit. Care Med. 192(7), 826–35 (2015).