Liquid-like droplets organize the genome
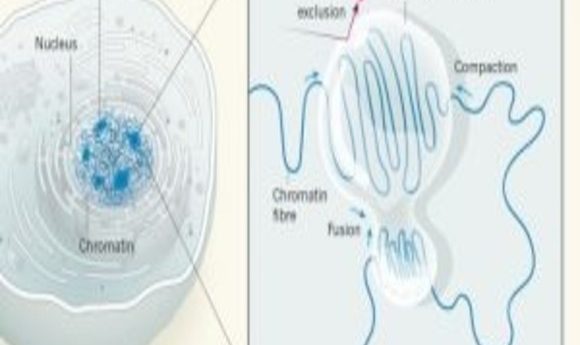
Two recent studies show that heterochromatin domain formation is driven by phase separation—a process in which proteins self-organize into liquid-like droplets that grow and fuse, allowing certain molecules to become concentrated while excluding others.
Diagram of chromatin compaction in liquid droplets (1).
The human genome consists of a two-meter-long DNA strand packed into a nucleus with a width of only about 10 micrometers. This feat is accomplished with the help of chromatin—a complex of DNA and proteins that forms chromosomes within the nuclei of eukaryotic cells. Densely packed heterochromatin is inaccessible to transcription factors and contains unused genes kept in a repressed state. This ensures the stability of the genome and helps cells to become specialized by preventing unwanted transcription [1].
Despite the high level of compaction, heterochromatin domains must remain accessible to enzymes to allow critical processes such as the efficient repair of damaged DNA. The compaction of DNA into heterochromatin is mediated by a protein called heterochromatin protein 1 (HP1). But it has not been entirely clear how distinct regions of chromatin can organize into large, condensed heterochromatin domains while remaining accessible.
Two studies published online in Nature shed new light on this question as advanced microscopy techniques reveal that heterochromatin domain formation is driven by phase separation—a process in which proteins self-organize into liquid-like droplets that grow and fuse, allowing certain molecules to become concentrated while excluding others.
In one study, Gary Karpen of Lawrence Berkeley National Laboratory and his team found that heterochromatin domains labeled with a form of HP1 called HP1a bear the hallmarks of phase-separated compartments in early-stage fruit-fly embryos and that purified HP1a phase separates in solution, forming liquid-like drops [2]. In an independent study, Geeta Narlikar of the University of California, San Francisco, and her team discovered that a human form of HP1 called HP1α can spontaneously phase separate in solution and form liquid-like droplets and that known components of heterochromatin preferentially partition into the HP1α droplets [3].
“Segregation of the genome into active and repressed states has been observed for decades, and the central role of heterochromatin in sequestering regions of the genome from transcription has also been well-known,” Narlikar said. “What this work suggests is that the functional segregation may be enabled in part through physical segregation in a phase-separated droplet-like state, like oil in water. A membrane-less compartment could also help explain the selectivity of what is included or excluded from a chromatin domain.”
The two studies offer a fundamentally different perspective on heterochromatin formation, providing new opportunities for understanding how architectural and biophysical properties influence chromatin domain formation and overall genome function. For example, the dynamic properties of a liquid-like domain might facilitate the repair of DNA breaks within heterochromatin [1]. Liquidity would allow relocation of the damaged DNA segment from the center to the boundary, where it can be accessed by the large protein complexes required for repair. Complexes that mediate transcription or replication could also access heterochromatic regions in this way, allowing them to act at the appropriate times without disrupting the domain as a whole.
“The topic of phase separation in biology is growing in popularity and has emerged as a very informative new perspective for understanding long-standing biological questions,” said Amy Strom, a graduate student in the Karpen lab and first author of one of the studies. “Interdisciplinary approaches like using physics to study biology have lent fresh ideas to problems that have gone unanswered for years, and we are excited to apply these ideas in the field of chromatin regulation.”
A Fresh Perspective
In their new study, Karpen and his team performed high-resolution 4-D analysis using lattice light-sheet microscopy—a technique that allows imaging of anything from molecules to embryos at high spatiotemporal resolution. To assess the movement of fluorescently tagged HP1a near the heterochromatin domain boundary, they used raster image correlation spectroscopy. They also used a fluctuation correlation spectroscopy derivative called number and brightness analysis to identify subcellular regions in which fluorescently tagged proteins underwent coordinated movement.
“These techniques allowed us to see that heterochromatic proteins were really contained by a liquid–liquid barrier, similar to an oil–water interface that keeps a water-soluble dye specifically in the water compartment,” Strom said. “However, these techniques only measure net movement of many proteins at once, so we’re working on tracking single molecules near that interface in the future to confirm that this is really happening at the level of individual proteins.”
The researchers showed that the fruit-fly HP1a protein undergoes liquid–liquid demixing in vitro and nucleates into foci that display liquid properties during the first stages of heterochromatin domain formation in early fruit-fly embryos. Furthermore, in both fruit-fly and mammalian cells, heterochromatin domains exhibited dynamics characteristic of liquid phase separation. The domains grew by fusing together, they repelled large, inert macromolecules, and they mostly dissolved in the presence of an alcohol that disrupts weak hydrophobic interactions.
“This study is important because it changes our perspective on the way we think about DNA organization in the nucleus. Basic physical principles are at play here, and it means we can understand and manipulate genome organization through very different mechanisms than previously,” Strom said. “Phase-separated systems are very sensitive to things like pH, temperature, and salt concentration, so it’s an elegant way that the cell can organize many regions of DNA at once and react quickly to a change in its environment.”
Based on these findings, the authors propose that emergent biophysical properties associated with phase-separated systems are critical to understanding the unusual behaviors of heterochromatin and how chromatin domains in general regulate essential nuclear functions. With respect to regulatory mechanisms, selective permeability imparted by a phase boundary provides an alternative mechanism to chromatin compaction for controlling fundamental heterochromatic functions such as transcriptional silencing, replication, and prevention of aberrant DNA damage repair.
“We’re interested in how this knowledge of phase separation can help us understand heterochromatic function and dysfunction better—this nuclear domain is involved in aging and longevity, and mutations in certain heterochromatic proteins cause progeria, or early-onset aging diseases,” Strom said. “Where previously it was very inefficient to target things to heterochromatic areas, this knowledge about the organization of heterochromatin essentially could allow us to send tools, proteins, and therapies specifically to heterochromatic sequences.”
A Serendipitous Discovery
A hallmark of heterochromatin is its ability to spread to adjacent regions and cause gene silencing. Aberrant heterochromatin spread is thought to promote cancer by permanently repressing genes required for normal differentiation. “One of our long-term goals has been to uncover the biochemical capabilities of heterochromatin molecules such as HP1 so we can understand how heterochromatin is regulated in vivo,” Narlikar said. “We hope that by understanding basic mechanisms, we can better understand how aberrant heterochromatin formation leads to diseases like cancer.”
In the Narlikar lab, the discovery that the human HP1α protein can form phase-separated droplets arose through a fortuitous observation. Inspired in part by recent work showing that phosphorylation of the N-terminal extension of human HP1α is important for the formation of heterochromatin foci in cells, the researchers generated different types of phosphorylated HP1α proteins to look for additional effects. While working with one phosphorylated version of HP1α, they observed the formation of a turbid solution upon cooling the protein on ice. Investigation of the turbid material under a microscope revealed liquid droplets.
“We speculate that others may have seen the cloudy precipitate-like material earlier but perhaps dismissed it as aggregation,” Narlikar said. “For us, the context was different due to the accumulating studies on phase separation in other systems. These studies allowed us to imagine similar possibilities in a chromatin context.”
Further investigation revealed that either phosphorylation of the N-terminal extension of HP1α or DNA binding promoted the formation of phase-separated droplets. Using a sophisticated in vitro assay, the group showed that liquid-like drops of HP1α can form on a stretched DNA strand and pack it together into a small, dense point. “The application of DNA curtains technology allowed us to visualize the compaction of DNA by HP1 in real time,” Narlikar said.
The group also demonstrated that DNA wrapped around histones carrying chemical modifications characteristic of heterochromatin domains becomes locally concentrated through enrichment inside an HP1α drop. These findings suggest that heterochromatin-mediated gene silencing may occur in part through sequestration of compacted chromatin in phase-separated HP1 droplets.
“The discovery that proteins can undergo liquid–liquid de-mixing to form droplets is quite recent,” said Adam Klosin, a postdoctoral researcher in Anthony Hyman’s lab at the Max Planck Institute of Molecular Cell Biology and Genetics; the duo co-authored an accompanying News & Views article published in Nature [1]. “Biologists simply did not think of proteins in these terms. The classical key-lock mechanism of interactions is still the prevailing way of thinking about intermolecular interactions.”
According to the authors, the findings also suggest that the two different drivers of phase separation, DNA binding and N-terminal extension phosphorylation, could provide different means of regulating heterochromatin. For example, phase separation coupled to DNA binding may be used when rapid chromatin compaction is needed, whereas N-terminal extension phosphorylation may provide a means to regulate the size of heterochromatin bodies because phosphorylation enables large-scale assemblies without DNA binding.
“The work provides a new perspective on a long-studied process that is central to regulating cell identity and development,” Narlikar said. “[It] shows how fundamental chemical and physical principles can help us understand complex biological phenomenon and emphasizes the importance of curiosity-driven basic research.”