A multiomic platform to further cancer research
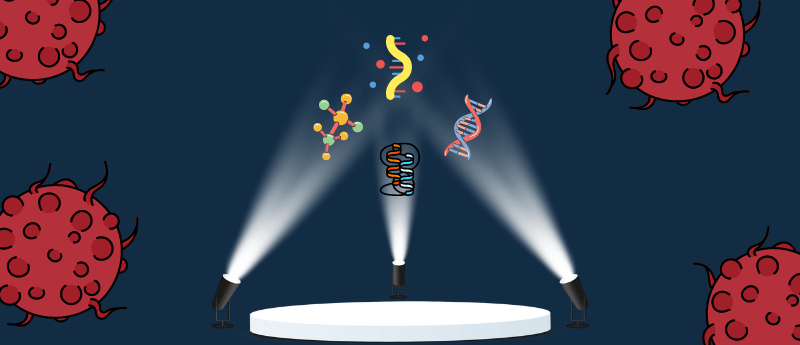
Shawn Levy (left) is Chief Scientific Officer and Senior Vice President of Applications at Element Biosciences (CA, USA), leading a multidisciplinary team supporting applications development and external partnerships and collaborations. In this interview from AACR 2024, Shawn discusses their new platform, AVITI24, which combines sequencing with multiomic analysis to give deeper insights into cancer biology.
What are you presenting at AACR?
We’re presenting how we can dramatically accelerate the understanding of complex biological samples through the use of multiomics, and also announcing the expansion of our sequencing instrument to perform multiomic analysis, as well as sequencing. By multiomics, we mean the ability to simultaneously profile DNA, RNA, protein – including some forms of phosphorylated versus unphosphorylated protein – and cell morphology. On a single platform, you can do these assays either individually or in combination, depending on what the experimental design would benefit from.
Can you tell us the working principle of this instrument?
At Element Biosciences, we developed a sequencing chemistry that we call avidity base chemistry or ABC, and this is an extension of that. We’re still leveraging the same optical and fluidic systems, but we’re using a different flow cell that takes advantage of the flexibility in our surface chemistries. Because that surface chemistry is flexible, we’re able to bind cells and then detect the analytes of interest – RNA, protein, etc. – via a direct probing mechanism. For example, for RNA, it’s an oligonucleotide probe for proteins and antibodies. Then we use ABC chemistry to detect what probe is bound.
By doing that across the entire flow cell, we can either do multiple individual experiments, up to 12 individual wells per flow cell, or just an entire flow cell, where you can profile 1–2 million cells, depending on confluency and cell size. That allows you to then aggregate all of that data on an individual cell basis, with sub-cellular resolution. We’ve been able to leverage that ABC chemistry in a unique way so that we can detect up to 3000 individual transcripts in the same cell.
What are some of the key benefits of being able to combine these two applications into one machine?
There are several, but a key one is that in complex biology, when you think of the central dogma of DNA to RNA to protein, most technologies allow you to look at, especially in a high-throughput manner, only one of those analytes or another. For example, mass spectrometry and proteomics, sequencing and genomics. To profile them simultaneously or to at least integrate the data, you usually have to use multiple instruments and multiple platforms, and therefore, have to split samples.
We’re introducing the ability to take a single sample and look at multiple analytes simultaneously. This develops a better picture of what’s going on, where you’re not just looking at one step in the biological process, but you’re able to profile several steps.
What are some applications of this technology in cancer research that you’re particularly excited about?
This technology has many applications in cancer research. It’s useful for exploring the complexities of cancer biology and investigating therapeutic response and resistance; a critical aspect of cancer research is understanding why some patients respond and others don’t. This technology is also good for human immune profiling, understanding the role the immune system plays in cancer progression, and how we can leverage it to treat cancer. Beyond that, in drug development and other screens where we’re looking at toxicity or other responses.
Hunting for oncopathogens with multiomic approaches
Here, Jason Yongsheng Chan discusses his recent presentation, “Multiomics approaches for novel oncopathogen discovery and related mechanisms” at AACR 2022 and his key takeaways from the conference.
Since this is a cell-based assay, and you can look at primary cells, we have the opportunity now to look at patient dynamics in a high throughput, very rapid way. I think that one of the differentiating features of this technology is that, as I mentioned, on an individual flow cell, we can run 12 different conditions simultaneously. This allows you to do either multiple patients, multiple time points or multiple conditions on the same patient. Because we have two flow cells, you can essentially run up to 24 samples at the same time, and with a 24-hour turnaround time, you can very quickly and very iteratively go through large numbers of samples in a very efficient way.
Do you have any key tips for utilizing this instrument?
Now that technologies are getting more advanced in terms of their sensitivity, it’s highlighting a few things. One is that sample preparation and sample metadata are becoming even more important. It’s a critical aspect of the success of these types of biological experiments, where you have to really understand what’s going into the technology to understand what’s coming out.
We’re focusing on taking a stepwise fashion in terms of the types of samples and the types of materials we’re inputting, which is why we’re focusing on cells. I think one of our common questions is: can we do more things? Can there be a greater diversification? And certainly, the future holds great promise in that. But we wanted to bring forward a technology under a set of conditions that we could support robustly, and that’s what we’ve done in this case.
So, from a best practices perspective, having high-quality samples equals high-quality data. We’re taking a holistic approach to how we’re developing protocols for the platform so that we can give strong guidance when someone gets started with it, they’re not just starting from zero, they’re starting from an educated perspective.
What impact do you hope this instrument will have on the cancer research field?
I think in cancer research over the last 20–25 years, there has been the expectation that if we understood that single signature, whether it be DNA or RNA – DNA from a mutational perspective, RNA from a transcriptional perspective – then we’d be able to make these massive inroads into detecting and treating cancer. I think that while we’ve certainly made progress, that progress has not been as complete as everyone had hoped.
With this technology, we’re hoping to bring forward additional resolution, the ability to look at DNA, RNA and protein, even different forms of the proteins, at the same time, and do cellular perturbations to understand some of the mechanistic effects to try to drive our understanding. Not only the understanding of the basic biology of cancer, but the understanding of the dynamics of cancer, and we think that’s critical.
We’ve known for a while now that cancer is a disease of genomic instability; it is generally rooted in a DNA repair defect. But once you have that DNA repair defect, the evolution of cancer is very personal. When you look at trial data from some of the early detection or multi-cancer detection technologies that are out there, they’ve shown great promise and success in around 70% or 80% of patients. But it’s not 90+%, which means there’s still a fraction of these patient samples and biology that we don’t understand. Our hypothesis is that if we can bring together tools that allow perturbations to be measured and tools that allow higher resolution analysis of these samples, we can close that gap.
The opinions expressed in this interview are those of the interviewee and do not necessarily reflect the views of BioTechniques or Taylor & Francis Group.