Pick of the posters: Neuroscience 2022
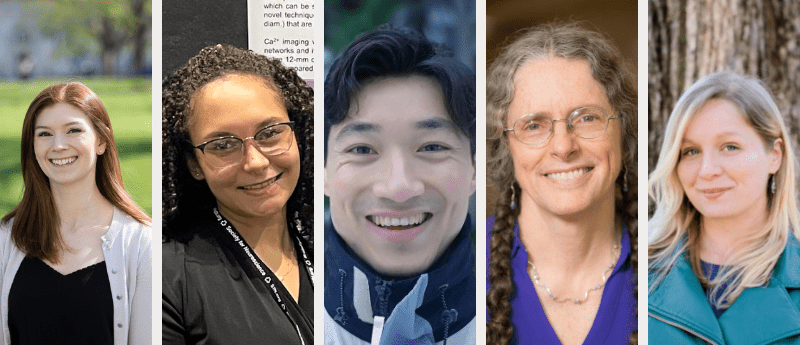
At this year’s Neuroscience 2022 (San Diego, CA, USA; 12-16 November), the annual meeting of the Society for Neuroscience, we took a trip around the poster hall, picking out our favorites and those we thought you would most like to hear about.
Check out our top 5 poster selections below.
Nonlinear dynamics underlying epileptic seizures at single-cell resolution across the whole brain
Dominic Burrows
What techniques were used in the creation of your poster?
In terms of data generation, I was using a two-photon microscope to image larval zebrafish. We have some genetically encoded calcium reporters in the larval zebrafish, and they’re also transparent. That allows me to image at a single-cell resolution across the entire brain, so I have about 20 to 30,000 neurons. And then I’ve applied a variety of different computational methods to understand the dynamics that allow seizures to occur at the whole brain level.
What are some of the key takeaways from your poster?
I think one of the most interesting things is that people often think about epilepsy and seizures as these very synchronous brain events where you have different neurons and different brain areas working in concert together. And one of the key findings of my work is that actually, while this is true, it’s not the entire picture because there is a lot of neuronal activity that is highly nonlinear, so not correlated, but highly complex.
Essentially, at the microscale, when you look at seizures at the single-cell level, the network interactions that allow them to occur are very different from what you see at the EEG level, where things look very linear and very correlated. We’re investigating this difference and the underlying mechanisms that account for it.
Part of the problem with higher scale, EEG work is that you’re averaging across a lot of activity and non-synchronous behavior. At the single-cell level, you can really see what is every single one you’re on doing. You obviously have different cell types, and they’re behaving in different ways, so that’s been really key in understanding this.
Any tips for best practice when using the techniques employed in your study?
I’m a computationalist mainly, and I work with a lot of dynamical systems techniques and generative models. I think if you’re using these techniques alongside empirical data, you have to make sure that the different sides of your work, the experimental side and the computational side, feed off each other. Start off with some experiments and use them to inform your models. But then you also need to validate your models by looking at whether your predictions hold true in the data. Going back and forth between the bench and the computer is very important.
High-density recordings for long-term electrophysiology in developing brain organoids with automated fluidics platform integration
Jess Sevetson
The Braingeneers, University of California, Santa Cruz (CA, USA)
What techniques were used in the creation of your poster?
We use electrophysiology on a high-density multi-electrode array, as well as a number of engineering and computational techniques, fluid force modeling and measurements of various physiological conditions.
What were the key takeaways from your poster?
We wanted to communicate that we are in the process of developing an open-source reproducible microfluidic platform that will allow you to feed your organoids and keep them in a constant state of metabolic health, while also imaging them from overhead and conducting electrophysiological recordings. We’re integrating a lot of different modalities so that you can analyze your organoids holistically.
What do you think the key impacts of your poster will be on the field?
I think this is going to allow people to collect more data in more ways. Also, the Internet of Things architecture that we use to get all these systems to talk to each other is very good for setting up experiments remotely. In my opinion, this will contribute to the democratization of science and allow people from spaces that don’t necessarily have access to all the tools, to actually do this work.
Integrated proteomics to map the tau interacting partners in Alzheimer’s disease
Sarah Shapley
Seyfried lab, Emory University (GA, USA).
What techniques were used in the creation of your poster?
I’ve mostly just been using in vitro techniques with Human Embryonic Kidney (HEK) cells. We’ve created a Tau construct that allows us to pull out its interactors upon multimerizing. Through affinity purification, mass spectrometry-based proteomics, and western blotting we can identify and validate these interactors.
What are the key takeaways from your poster?
I’m currently working on a project to understand more about Tau interactors in the context of disease. I’ve generated this construct analogous to the HEK-FRET tau aggregation biosensor cell model system that uses the 4R microtubule-binding region of Tau. Our construct also leverages a proximity labeling technique TURBO ID, which uses the split TURBO biotin ligase fragments and was developed out of Alice Ting’s (Stanford, CA USA) lab. These fragments are composed of a nonfunctional enzyme when apart but when recombined, they tag proximal interactors with biotin within a 10-nm range.
We’ve been enriching the biotin-tagged proteins and identifying them using mass spectrometry, to identify proteins that potentially have some impact on the modification of Tau aggregation. We found from our HEK cell proteomics data that approximately 34% of these proteins overlapped with tau-interacting proteins immunoprecipitated from Alzheimer’s disease brain lysates. And so we’re still optimizing, but leveraging this data to go into more disease-relevant model systems, such as neuronal cell lines, and hopefully lending some more insight into oligomeric Tau interactors specifically.
What do you think the key impacts of your poster will be on the field?
In the nanosymposia for the Tau at SfN, there was a lot of focus on the toxicity of soluble Tau species and I believe this novel split Turbo Tau cell model will allow us to define new interactors of Tau and their role in disease as potential therapeutic targets. Ultimately, this model allows us to study disease relevance of multimeric Tau interactors, which can lend mechanistic insight into what promotes Tau aggregation diseases.
Dietary diversity correlates with modulatory capacity in three species of majoid crabs
Patsy Dickinson
Bowdoin College (ME, USA)
What techniques were used to generate the data for this poster?
In our research, we mostly use physiological techniques, and for this project, we used extracellular electrophysiological recordings. To do this, we dissect out the nervous system and put extracellular electrodes on to record activity in the motor nerves. This particular system is useful as the motor nerves are fairly small and only have a few axons, so we can easily identify them and see whether they respond to the different peptides that we superfuse over them.
For the heart recordings, we took force transducer recordings of the entire heart. We can cannulate it and hook a transducer on it and look at the actual movements of the whole heart.
What were the key takeaways from your poster?
Our poster looked at the diversity of modulators; one question that has been asked for ages is why there are so many modulators. I work on the lobster or crab cardiac system and the stomatogastric system, which is a neural network that controls the motion of the gut and foregut. The network acts as a central pattern generator, which produces rhythmic patterns of activity in the absence of sensory feedback. The crab stomatogastric system generates a couple of different rhythm patterns that are flexible, so they generate different modes of that pattern. There are around 50 different modulators that have been identified that change the pattern and alter it. The question is: why there are so many?
The hypothesis has always been that it’s because they need to have many patterns to be able to eat different kinds of foods; however, there was not a lot of evidence for this. To investigate this, we looked at three species of crab that eat different food: one eats just kelp, and the other two are opportunistic feeders that eat anything. We looked at how the crabs responded to different modulators and the crabs’ modulatory capacity, which is the ability of the nervous system to respond to different numbers of modulators. We found that the crab that eats only kelp responds to fewer modulators than the other two closely related species.
We did a comparison with the cardiac system, which of course should have no relationship to whether they eat different kinds of food, and we found there to be no relationship. This suggests that one of the driving forces of modulation is the evolutionary need for flexibility.
What do you think the impact of these posters will be on the field?
We now have some evidence that evolution has in fact evolved modulation as a mechanism for developing flexibility. I think of it as an evolutionary substrate of flexibility, as you can have flexibility by changing the nervous system, but you can also have flexibility by changing the way that it responds to different modulators. The extent to which it’s modulated is a mechanism to allow flexibility evolutionarily, which is not something that we’ve really thought of before.
A novel technique for creating small neuronal networks in vitro
Nicole Diaz
College of New Jersey (NJ, USA)
Can you tell us a bit more about this technique?
It’s a very simple and robust technique to make mm-sized neuronal networks. We used the tool to cut out a 3×3 array of 1mm diameter holes in a very thin sheet of silicone membrane. We then placed this sheet over a glass cover slip in order to mask a cytophilic protein coating needed for cell adhesion to the glass. After one day, the mask is removed leaving behind small neuronal networks in the regions where there were holes and glass had been exposed. These regions are small enough for our optical system to capture an entire neuronal network within the field of view (FOV).
Having small networks eliminates a previous problem associated with our goal to understand network bursting activity based on connectivity, which was measured using an apparatus that combined calcium imaging with single-cell laser scanning photostimulation. Network bursting is characterized as synchronized neuronal activity consisting of a train of action potentials, which is similar to brain wave activity that occurs during sleep. How and when network bursting occurs is something that we don’t really quite understand.
Previously, our investigation was limited by the FOV, which is much smaller than the actual size of the network, making it difficult to draw any conclusions about the network at large with any degree of confidence. Thus these small networks allow you to study the connectivity and activity more accurately.
Are there any insights that you’ve gained from using this technique so far?
When you measure the bursting activity of these small neuronal networks, which are 1 mm in diameter, versus the large neuronal networks, which are 12 mm in diameter and cover the entire coverslip, you see that the frequency of the bursting activity of the large networks is consistently higher. Additionally, you also see that younger cultures are more active in large networks than small networks. What this suggests is that long-range connectivity of neurons plays an important role in early network development, for setting up the desired connectivity pattern for bursting to occur.
Another feature of smaller networks is that the neurons have a tendency to cluster together. This is not desirable because these clusters are so interconnected that they essentially act as a single cell. thus decreasing your overall cell density.