The integrative approach to studying information processing in the brain
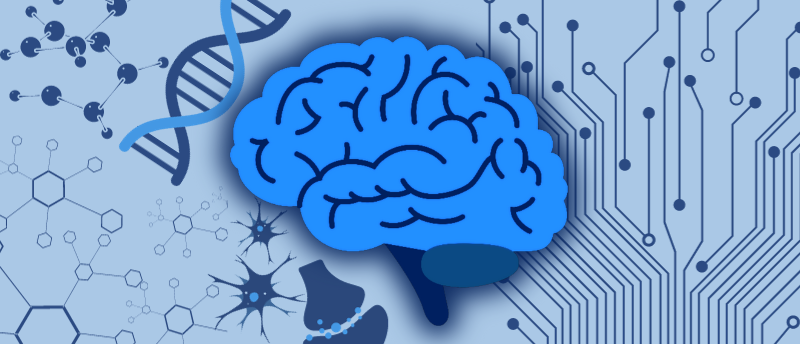
André Fenton (left) is the Director of the Center for Neural Science in the Faculty of Arts and Science at New York University (NY, USA) researching how the brain processes information and stores memories using an integrative approach.
With a background in systems neuroscience and using in vivo electrophysiology to explore the signal properties of place cells – neurons in the hippocampus activated by the entry of the organism into a specific location in its local environment – Fenton continues to use these cells in his current research as canonical cognitive signals in the brain. However, since his training, Fenton has adopted the attitude that one can’t understand the brain very well using systems neuroscience without paying close attention to cellular and molecular neuroscience. Therefore, Fenton characterizes himself as an integrative neuroscientist who uses every tool available to him to try and establish a multidimensional understanding of the nervous system’s function and computation.
Digital Editor Annie Coulson spoke with Fenton about his research and the importance of integrative approaches to neuroscience at the Society for Neuroscience’s (SfN) annual meeting, Neuroscience 2023 (Washington, DC, USA, 11–15 November), where he provided insightful summaries of his posters at the event and answered some key questions regarding his work.
DNA expression in fragile X syndrome
I’ll start by highlighting our poster presenting an examination of DNA expression in a mouse model of fragile X syndrome. Fragile X syndrome is the most common single gene mutation determinant of intellectual disability and autism features. The molecule silenced in the syndrome – or knocked out in the mutant mouse – is an RNA-binding protein that presumably affects how translation of RNA into protein operates. So, this poster examined that mutant model’s DNA expression upstream of translation. There are quite a few changes in DNA expression that we were able to characterize, and some of them affected the manufacture of plasticity proteins, one in particular called PKMζ. Fundamentally, this poster highlights that known changes in translation cause feedback alterations in the transcription of the DNA into RNA, which serves as the instructions for protein manufacture.
Another poster displayed the systems physiology in this mutant model, which showed that despite lots of unusual or aberrant physiological expressions at the level of synaptic plasticity, coordination of neural spike trains and local field potential oscillations, the animals had very mild behavioral deficits in a variety of cognitive tests. I wouldn’t even characterize these as deficits, really, because the mutant mice didn’t perform the tasks wrong, they just performed them in different, slightly unusual ways. The animals are just finding different solutions to the same problem, probably because they have different physiology. This provides another example of feedback and compensation in this system.
Proteomics for PKMζ
We also had several posters on the molecular biology of PKMζ and its interaction with the structural synaptic protein KIBRA. PKMζ is one of the only molecules known to be essential for the maintenance of long-term potentiation (a cellular model for studying memory). Once you establish synaptic plasticity strengthening, there needs to be a mechanism that can maintain that strength over long periods of time to establish long-term memory.
How do you, in a protein synthesis-dependent way, maintain those synaptic changes with proteins that turn over every few days? The proteins don’t last forever; they get made and then they get destroyed and replaced. Therefore, there’s no obvious way to maintain that protein synthesis-dependent expression and synaptic plasticity. We presented the first work showing how that can be done and it’s because of an interaction between a structural protein called KIBRA and the protein kinase PKMζ. Because of their interaction, they form a complex that is more stable than either of the molecules on their own. Once they form that complex, although it’s in a dynamic equilibrium, the complex itself is more stable and so it aggregates and persists there in the complex form, despite the turnover.
Hippocampal neural cell biology
The last poster that I’ll mention looked at how the hippocampal neurons as well as neurons in an indirectly connected area, the anterior cingulate cortex, fluctuate in their information processing during a place avoidance task. The place avoidance task is designed so that animals can recognize two different interpretations of where they are. In this task, animals in a slowly rotating arena are able to recognize where they are in the rotating frame of the arena as well as where they are in the stationary room. Over the last few decades, we’ve shown that hippocampal neurons alternate between coding where the animal is in the stationary room versus where the animal is on the rotating arena. We were surprised to find very similar activity patterns in the anterior cingulate cortex and the hippocampus, even though these two areas are not directly connected to each other. This begs the question of how they’re coordinated with each other so tightly given their relative neural circuitry independence.
What you can recognize from these posters is a general theme of neurobiological mechanisms for information storage and information processing that have a subjective quality to them. The animal can, at one moment, care about where it is in the room or care about where it is on the floor. Additionally, all the feedback mechanisms I’ve described at the level of neural systems as well as the level of molecular biology provide for there being internal mechanisms rather than externally driven mechanisms that make these adjustments.
What was your main presentation at SfN 2023 regarding?
Here, I discussed the beginning of my intellectual journey, which involved looking at how external features of the world cause neurons to fire so that we can describe their tuning according to external features of the world. I recognized early on – but did not know how to deal with – the noisiness of the neural signal from that point of view; I found numerous deviations from what you would expect from the hypothesis that place cells or head direction cells are driven by the actual external cues in the world or the actual directional cues that are stable in the world and useful for navigation. My lecture outlined those findings that were inconsistent with that externally driven set of activity patterns.
What we adopted instead was a different point of view; that it’s very possible the activity is internally organized rather than allocentrically organized by stimuli from the external world. It turns out that when you adopt that point of view and you ignore the external world, the cells are internally organized very strongly. In fact, they organize the activity better than the noisy external features that were described. However, you still must engage in the world and show up somewhere at a particular time and place. So how does the internal organization translate to the outside world? The answer seems to be in how this internal activity is associated with the world through synaptic plasticity mechanisms. The mechanisms aren’t organizing the internal activity, but they are registering that activity, which explains why you can have a subjective view of something.
My special lecture highlighted that the PKMζ–KIBRA synaptic plasticity mechanism was one of the mechanisms that allows the reliable attachment of these internally organized activity patterns to the external world.
What are some of the key techniques and technologies that you use in your research?
I do everything I can to be integrative and to work across all levels of biology because I understand that they are interacting. Therefore, my list of technologies is very long. We use modern electrophysiology, such as neuropixels and silicone probe recording techniques. We also use miniature-microscope calcium-imaging techniques, which involve a viral transfection of neurons of interest to get cell-type-specific expression of those fluorophores or the calcium-responsive fluorophores. We use several computational techniques to look at the manifold structure or the population geometry, which are the internal or neurometric methods. Of course, we employ various kinds of biochemistry methods – proximity ligase assays, for example – to demonstrate that KIBRA and PKMζ are interacting, not just co-localizing.
We also use genetic techniques, such as pharmacogenetics, to knock out various components. We needed to develop multiple ways of interfering with the PKMζ–KIBRA interaction so that we could come to a convergent conclusion when no single experiment was perfect. Obviously, we use RNA-sequencing and variants of RNA-sequencing to measure the transcriptomes and various bioinformatics techniques to analyze those transcriptomes.
As I mentioned above, we often use manifolds, which are low-dimensional subspaces in which the activity of multiple neurons firing in unison can be described mathematically. This technique can also illuminate the molecular expression within a cell. If you teach a mouse to learn something – and therefore teach a neuron or a set of neurons to learn something – you are looking at changed gene expression. One possibility is that the 20,000 genes all change independently of each other. And another, more realistic possibility, is that the 20,000 genes break off into little subgroups that co-vary in their response to the training and their response to, for example, synaptic plasticity mechanisms or memory consolidation. Therefore, instead of following a single molecule and saying this molecule is important for this process, we’re using systems techniques to try to understand the manifold organization or the co-relationships amongst diverse molecules that together can accomplish the same output consequences of the biochemistry instead of all acting independently or by serial mechanisms.
What are the biggest challenges that you face in your research?
I think one of the biggest challenges is to convince my colleagues that an integrative view of the nervous system is feasible. Humanity will benefit when we’re able to provide that, but very few people are trained to do this kind of work. I’m certainly not trained to do it, although pioneers aren’t trained in anything they pioneer. We haven’t worked out the perfect ways in which to do the integration. We are trying to figure that out, which sounds like a very abstract and maybe unsolvable problem, but I’m an optimist! I think if you look at our work, you can see that we’re making progress. We don’t yet have the perfect framework to offer to the neuroscience community in a neat package, but we are working on it. That’s the hard part.
What are the translational aspects of your research?
I believe that one of the most valuable things we can do as a community, and even as individuals, is impactful science. By impact what I mean is to provide science that allows people who are not scientists to have better lives. Therefore, how we use these concepts is important, which is why I do my very best to communicate complicated concepts as simply as I can. The hope is that we will help psychiatrists and other clinical practitioners and drug developers use these concepts so that they can translate the findings that they might generate in their own research within a proper conceptual framework. This framework would be an integrative framework, where various levels of biology interact with each other. This sort of framework provides feedback, which forces you to think of things in terms of networks and to look for networks’ states of equilibrium rather than simply a hierarchical process.
Looking to the future, what is the focus of your research?
My focus right now is to do what I call single-subject experiments, which we have been trying to pioneer. In these experiments, all the techniques I just described are applied to the same subject to generate massive datasets that look at a moment of learning, for example, or a moment of recollection, from all of those different levels of biology. Why is the same subject important? Because if you commit to what I have argued in my special lecture that these processes are internally organized rather than externally organized, then each subject may have an idiosyncratic way of solving that problem today. And tomorrow, they might have a different idiosyncratic way of solving that problem. No one behaves exactly the same way every day. You make different choices.
Given that there are different internal cognitive processes that can always accomplish the same goal when looked at from the outside, we feel that it’s necessary to record and measure from the transcriptome to the synaptic physiology to the behavior in the same subject because those different levels of biology are likely to be interacting with each other, constraining the solutions so that they are related to each other for today’s particular outcome. This is a challenge that we have in large part overcome by figuring out how to collect that data. I’m starting several collaborations with data scientists and machine learning specialists as the problem we’re having is working out how to integrate those different datasets into a meaningful understanding rather than a description of the data.
The opinions expressed in this interview are those of the author and do not necessarily reflect the views of BioTechniques or Taylor & Francis Group.