A peek inside a single cell
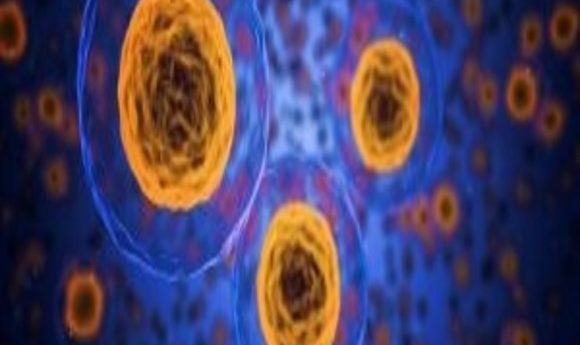
More advanced techniques and assays for simultaneous analysis of DNA, RNA, and proteins from single cells are starting to emerge. But just what information will be gleaned from such diverse datasets?
Today, new technologies and instruments are being designed to generate the highest throughput possible in the shortest amount of time, opening doors for researchers to ask questions that just 5 years ago would have been unthinkable. Now, our scientific culture is adopting a “bigger is better” attitude.
In the past, researchers performed genome, transcriptome, and proteome studies using collections of millions or billions of heterogeneous cells. Single, individual cells—the most fundamental units of an organism—were too difficult to isolate alone. Even if it had been possible to isolate large numbers of single cells for analysis, scientists believed that one cell possessed too little starting material to effectively study.
In recent years, however, there has been both a conceptual and technical change in the way researchers approach single cells. Conceptually, scientists began to realize that individual cells possess unique signatures, phenotypes, and lineages—information that gets lost when studying cells en masse. Now, most researchers hold the opinion that studying single cells makes it possible to gain a deeper biological understanding of cell function, cell–cell interactions, and cell organization.
On the technology side, researchers initially focused on developing ways to simply isolate single cells, and then ultimately to obtain either DNA or RNA in sufficient quantities for studying the individual genomes or transcriptomes. Advances in flow cytometry and microfluidics, along with better genome-wide amplification technologies and sequencing methods, led to more single-cell studies emerging in the literature in recent years.
But scientists are not simply sitting back now and settling for single cell genome or transcriptome studies. Developers are looking to move further ahead toward more sophisticated approaches that are capable of simultaneously isolating and analyzing DNA, RNA, and even proteins from single cells. The objective now is to connect the dots between these biomolecules and, hopefully, arrive at an even more complete picture of how genotype influences phenotype in cells and beyond.
Insights From Many Biomolecules
For years, researchers sought out mutations in genomic DNA in an effort to identify molecular pathways involved in disease processes. Fundamentally, the idea makes a lot of sense; if there is a unique mutation in a key gene, that change is likely the cause, or one of the causes, of a particular disease phenotype. But in many instances, the relationship between a mutation and a phenotype is more complicated and often indirect. This realization is leading some researchers to develop newer methods for obtaining both genomic and transcriptomic data from the same single cell at the same time so that they can more closely study the impact those specific DNA changes have on global levels of RNA and protein production.
One of the first dual isolation strategies for single cells appeared in early 2015 when Alexander van Oudenaarden from the Hubrecht Institute-KNAW in the Netherlands and his colleagues presented the gDNA-mRNA sequencing (DR-seq) technique in Nature Biotechnology (1). In DR-seq, scientists lyse single cells and then perform reverse transcription. From there, they amplify both the genomic DNA and cDNA using a quasilinear amplification strategy. After amplification, they split the sample into two halves, one of which they use for further genomic DNA amplification and sequencing to analyze DNA, while the other is used for cDNA analysis to interrogate the cell’s transcriptome. The approach enables the direct comparison of a single cell’s DNA and RNA status at the same time. Using DR-seq, van Oudenaarden and his team showed correlations between transcript numbers and DNA copy numbers among different cells—a result that would not have been possible without using dual isolation.
One of the things that made the DR-seq approach so unique was that it required no physical separation of DNA or RNA. But following the publication of the DR-seq strategy, other researchers started to explore the possibility of physically separating the genomic DNA from RNA within a single cell.
Iain Macaulay from the Sanger Institute-EBI Single-Cell Genomics Centre in Hixton, UK and his colleagues described such a physical separation approach for simultaneous isolation of DNA and RNA from single cells in a 2015 Nature Methods article (2). In this case, the authors relied on biotinylated oligo-dT primers to separate and capture the cellular RNAs from the genomic DNA for analysis. Following the physical separation step, the team performed whole genome amplification followed by next-generation sequencing independently on both samples.
As Macaulay and his colleagues noted in their article, physical separation is most interesting because the approach lends itself to automation, paving the way for higher throughput single cell interrogation in the future. This is a crucial consideration for researchers interested in single cell studies. There are a large number of cells in most organisms, so being able to analyze tens of thousands of individual cells possessing unique phenotypes in a single experiment would provide important biological insights.
More recently in September 2017, researchers from Philips Research Laboratories in the Netherlands described how they constructed an on-chip assay for the differential extraction of DNA and RNA from single cells (3). Here, the researchers first sorted single cells and placed them into individual wells. Then they initiated a two stage lysis protocol: first, the cell membrane is lysed and RNA isolated, and then the nuclear membrane is lysed so that genomic DNA can be isolated. The entire protocol is run on a chip, making the approach highly automatable.
Even as more automated approaches to DNA and RNA extraction from single cells become available, developers are moving their sights toward incorporating proteins into single-cell analysis. In July, researchers at the New York Genome Center (NYGC) demonstrated a new technique capable of monitoring the status of 10 surface proteins while simultaneously measuring the transcriptome activity of more than 8,000 single cells.
“No other method allows simultaneous measurements of transcriptomes and proteins on the same scale,” noted Marlon Stoeckius, a research scientist at NYGC and one of the developers of the new technique, in a press release.
Stoeckius and his colleagues called their new approach CITE-seq, which is short for Cellular Indexing of Transcriptomes and Epitopes by sequencing (4). CITE-seq works by using antibodies that are labeled with oligonucleotides, making it possible to measure a cell’s proteins and RNA using a single cell readout.
With the growing number of protocols and assays enabling simultaneous extraction and analysis of genome, transcriptome, and proteome components on the rise, the next obvious question becomes, what will all of this new data tell us in the end?
Pulling the Data Together
Researchers and funding agencies are hoping that combining measurements of DNA, RNA, and proteins from single cells will promote development of new ways of looking at the molecular bases of many human diseases and their progression.
“One possible future direction is to use CITE-seq on tumor samples to examine both individual tumor cells and the different pools of immune cells that infiltrate the tumor. This approach could be very useful in the deep characterization of tumor heterogeneity and in the development of new immunotherapeutic approaches,” Stoeckius suggested.
To further advance development of single-cell analysis technologies and their applications, in February 2017, the National Institutes of Health (NIH) called for grant applications from small businesses developing new single-cell analysis tools.
In their funding announcement, NIH noted that, “The ability to measure genomic, epigenomic, transcriptomic, and metabolic status in individual cells [is] expected to provide new insight into molecular pathways in health and disease. In addition, powerful technologies are being developed to isolate and analyze rare cells from a heterogeneous population and to examine distinct cellular states in complex tissue environments.”
The extent of knowledge that will eventually emerge from looking at DNA, RNA, proteins, and metabolites in the same cell at the same time remains to be seen. But it is undeniable that scientists are on the verge of collecting large-scale, integrated datasets from individual cells for the first time. These datasets could eventually lead to cell atlases and maps that will go a long way toward explaining the molecular underpinnings of cell functions in larger structures, such as tissues or organs—something that only a couple of years ago would have been thought impossible.