Measuring activity in the developing brain
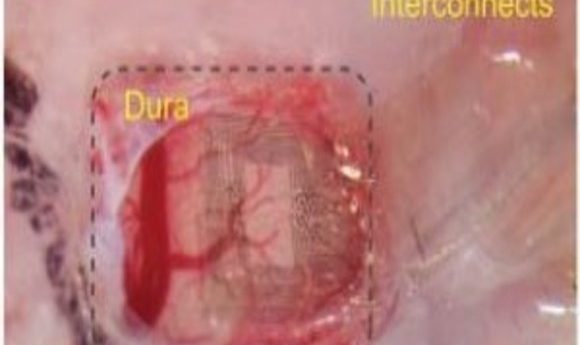
A new technique for recording electrophysiological activity in rodent neonates offers scientists an opportunity to explore the early developmental events that shape autism spectrum disorder and schizophrenia.
Neurodevelopmental disorders are more common than generally thought—and the associated costs are staggering. In fact, the Centers for Disease Control and Prevention (CDC) estimate that the United States spends somewhere between $11.5 and $60.9 billion each year to support children with autism spectrum disorder. The numbers for schizophrenia are worse; experts estimate the country-wide costs are somewhere in the range of $94 to $120 billion. And all of this pales compared to the life-altering effects these diseases have on the patients and their families.
According to Joshua Gordon, director of the National Institutes of Mental Health (NIMH), it is difficult to find effective treatments because of the dearth of knowledge regarding how these disorders develop over time. “We know from animal models of autism and schizophrenia that one of the main disturbances that we see in these disorders are alterations in connectivity between brain regions. We also see those same alterations in neuroimaging studies of patients with these disorders,” Gordon said. “But we have not yet been able to document when, during development, those alterations first appear—or what may be causing them. So, any tools that can allow us the ability to better investigate development could offer us new clues for where and when we should be looking for those disturbances.”
This need for new tools is one reason Gordon recently praised a new technology called the Neurogrid, a conformable surface electrode array that records electrophysiological patterns from the brains of rodent pups. The new recording device was presented at Neuroscience 2017, the annual meeting for the Society of Neuroscience—and Gordon spoke of it at length during the meeting’s National Institutes of Health press conference.
The Challenges of Studying Young Brains
Jennifer Gelinas, a pediatric neurologist at Columbia University Medical Center, was interested in finding a way to record electrophysiological activity in rodent neonate and pup brains. “If you’re trying to model a disease like childhood or infantile epilepsy, you really need a childhood or infantile animal model,” she explained. “The existing technology makes that very difficult. So I worked with my colleagues at [New York University Langone Medical Center] , Dion Khodagholy and Gyorgy Buzsáki, who had already developed the Neurogrid. Given its characteristics, we thought that this device would potentially be a perfect application to use in the developing brain.”
The Neurogrid is tiny, approximately 1 millimeter by 1 millimeter in size. Yet within that space, the device holds 120 electrodes patterned on a hexagonal lattice. Using an advanced nanofabrication technology, the researchers embedded those electrodes into a special substrate called Parylene C.
“Parylene C, which is very thin, only about four micrometers thick, is much like Saran Wrap,” said Gelinas. “And that’s kind of what it feels like. If you put the Neurogrid on your finger, it would have about the same consistency of Saran Wrap. That’s important because it can completely conform to the surface that you place it on, regardless of the shape of that surface. It also won’t put any pressure on the surface, which is why it’s so great for the developing brain.”
Gelinas and her colleagues tested the device by simultaneously recording from multiple cortical regions in both awake and sleeping mouse pups at different stages of development. They detected spontaneous network oscillations that changed at different ages, learning that precise temporal coordination of different cortical regions did not develop until the second week of life.
New Insights Into Normal Development
Currently, the Neurogrid records for 2-hour periods in different pups at different ages, but the researchers hope to extend the device’s capabilities to record activity over time in a single animal. While working on that, Gelinas also plans to explore normal physiological development of the cortex and cortical interactions.
“The next big step is to understand what might be going wrong in different disorders,” she said. “As someone interested in epilepsy, we could use Neurogrid to better understand what the critical time point is where the electrical activity of the brain in animal models of epilepsy starts to diverge from the normal activity we see in wild-type animals. And it’s the same for other neurodevelopmental disorders. Neurogrid could allow us to determine new electrical biomarkers for some of these disorders, but even more importantly, it can serve as a platform for determining some new systems-level therapeutic targets.”
Gordon agrees—which is why he is so excited about the possibilities concerning the Neurogrid technology. “The flexible nature of this recording device allows it to stay in the brain without doing damage as the animal develops. That’s wonderful in and of itself. But you can also study large areas of the brain at the same time,” he said. “While we know a fair amount of what goes on in individual brain regions as they develop, particularly the visual cortex, which has been so well studied, we don’t know much about how these different regions communicate with each other—which is what tends to go wrong in neurodevelopmental disorders. So if we can document when these alterations occur in development in animal models, we can get a clearer picture of when and where we should be looking in patients for those same disturbances and, with luck, find ways to intervene.”