PCR: celebrating 40 years of development
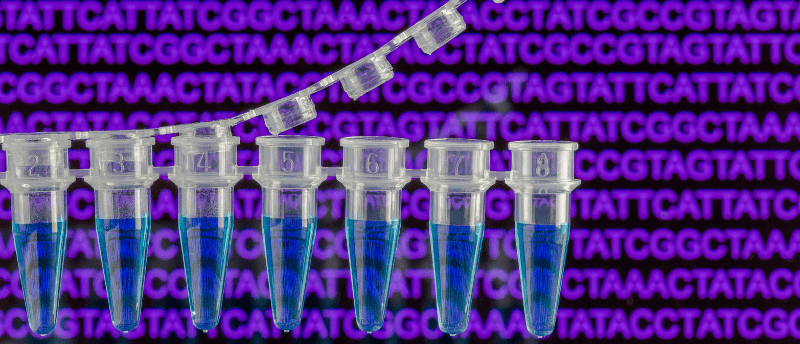
PCR is a technique used to amplify target DNA in a sample. It’s a well-known method that has undergone numerous modifications to enhance its capabilities. This year, it’s turning 40 years old.
PCR has been and continues to be employed throughout biological and genetic studies, from university labs and home test kits to hospitals and forensic labs. But how has the initial 1980s iteration of the method been modified to accommodate the needs of scientists today? Traditional PCR differs from more recently developed PCR techniques that can quantify different DNA segments within the same sample or amplify DNA from RNA segments. However, the basic principles of the method remain the same.
Here, we outline the key PCR developments that have emerged in the 40 years since its initial invention.
The original PCR
Kary Mullis is credited with inventing the PCR method in 1983 while working at the Cetus Corporation (CA, USA), winning the 1993 Nobel Prize in Chemistry for his ‘contributions to the development of methods within DNA-based chemistry’. Since its conception, PCR has been considered an important diagnostic and archaeogenetic method, providing an insight into viral discovery, hereditary diseases and ancient DNA [1].
PCR is a method that facilitates the amplification of a specific DNA segment – target DNA – within a sample. Once the sample is heated, the DNA strands separate, allowing two primers to bind to opposite DNA strands at the start of the target sequence. The primers direct where DNA polymerase should bind and begin to synthesize complimentary strands, extending the primer. Once the target DNA is replicated, the sample undergoes a heating step, separating the strands and allowing for another round of replication to take place. This cycle continues until the desired amount of target DNA has been replicated [1].
Using a thermostable DNA polymerase, named Taq polymerase, first isolated from Thermus aquaticus in 1976, researchers were able to streamline the amplification of targets; the thermostable polymerase is not denatured by the regular heating steps, so it can work throughout multiple replication cycles. Other thermostable DNA polymerases, such as Pfu polymerase, have also shown promise in PCR conditions [2].
This is the basic protocol upon which scientists have built adaptations of the method, refining PCR to meet their needs. From here, we have seen PCR grow into a method that can amplify and quantify multiple targets in the same well, be monitored in real time, use RNA as the starting material and/or use automation to make the process faster and more accurate.
Quantitative PCR (qPCR)
In 1993, Higuchi (Roche Molecular Systems, CA, USA) and colleagues built the foundation for the first quantitative iteration of the PCR method [3]. Real-time PCR, or qPCR, can be used to monitor the amplification of target DNA in real time, whereas traditional PCR cannot. qPCR is used to quantify the DNA segment of interest via the presence of a fluorescent dye, which can be tracked throughout PCR amplification. By determining the time point at which a significant increase in fluorescence is detected, it is possible to identify the starting copy number of the target in the sample [4] using the reaction cycle threshold and standard curve [5].
This makes it possible for researchers to monitor factors such as the prevalence of a species in an environmental sample or, if combined with reverse transcription, the rate of gene expression.
Reverse-Transcriptase PCR (RT-PCR)
Reverse transcriptase was discovered in 1970 by Howard Temin (University of Wisconsin–Madison, WI, USA) and David Baltimore (Salk Institute in La Jolla, CA, USA) [6]; because it is an RNA-dependent DNA polymerase enzyme, it was a natural alternative to the standard DNA polymerase used in the traditional PCR method and was therefore easily streamlined into PCR development. RT-PCR is an alternative PCR method that allows researchers to detect RNA in a sample, sequence the target RNA in a sample or conduct molecular cloning. It uses reverse transcriptase as a pre-treatment to transcribe RNA into cDNA, which can then be amplified via PCR for analysis. When combined with qPCR, RT-PCR can monitor the quantity of RNA in each cycle of amplification in real time. RT-PCR and qPCR methods are commonly combined to analyze viral RNA [7].
Hot-start PCR
With basic PCR and qPCR, researchers noticed that at lower temperatures, the primers added to the sample sometimes annealed to each other, creating a primer dimer that could then be replicated by DNA polymerase. This error interferes with the amplification of the target sequence. To combat the formation of primer dimers, premature DNA polymerase activation and nonspecific amplification, a method called hot-start PCR was developed in the late 1980s [8].
Hot-start PCR limits the reagents available to the sample at room temperature via molecular or chemical means until heating takes place, meaning that DNA polymerase is only functional after heating has removed the chemical or molecular block [2].
Multiplex PCR
In 1988, we were introduced to the concept of multiplexing [9], the capability to detect and amplify multiple DNA or RNA targets within the same sample using different primers for each target [10]. This technique requires the use of two or more distinct fluorophore probes that allow for simultaneous detection of the target sequences [10]. Multiplex PCR broadens the abilities of diagnostic tests as well as reduces cost. When first developed, multiplex PCR lacked the specificity and sensitivity necessary for accurate diagnosis. However, multiplexing has been improved since a comprehensive guide to primer selection and hot-start PCR were integrated into the technique [11].
Digital PCR (dPCR)
Although the concept of dPCR was first described in BioTechniques by Sykes (then Flinders Medical Center, South Australia) and colleagues in 1992 [12], the term ‘digital PCR’ was coined in 1999 [13]. dPCR has taken traditional PCR and qPCR to the next level, partitioning a sample into nanoliter-sized compartments to improve sensitivity and quantification accuracy. The random distribution of the sample means that the amount of target DNA or RNA in each compartment will vary. Following PCR in each compartment, the absorbance is measured to determine how much of a certain target DNA or RNA is in each compartment.
Scientists use Poisson statistics and the number of positive (compartments that contain one or more copies of the target DNA/RNA) reactions to determine the density and quantity of the target in the initial sample. Unlike qPCR, dPCR can do absolute quantification due to the segmentation of the sample, the assignment of positive and negative wells and the use of Poisson statistics to correct the target count in each positive well [5].
The future of PCR
PCR has established itself as an important technique with numerous applications in medicine, forensics and research. The last 40 years have demonstrated just how much the original PCR method can be modified in order to amplify and/or quantify the target genetic materials necessary for pathogen detection, hereditary disease diagnosis and biopharmaceutical production, for example. We can expect to see further modifications to the method, with digitalization and accessibility helping to further streamline analyses while maintaining and improving the sensitivity and specificity necessary for PCR’s wide range of applications.