The epigenetics of neuropsychiatric disorders
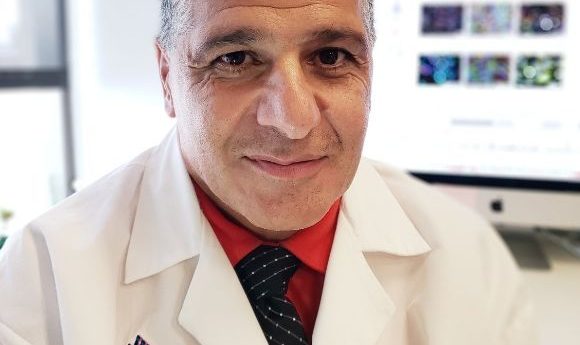
Schahram Akbarian discusses his work in neuropsychiatric epigenetics, the importance of understanding the 3D organization of the genome and his role in the PsychENCODE project.
Could you introduce yourself and give a background to your career?
I am a Professor of Psychiatry and Neuroscience at the Icahn School of Medicine at Mount Sinai (NY, USA). I trained in neuroscience and medicine in Europe and then, a quarter of a century ago, moved to the USA where, ever since, I have focused my studies on molecular changes in brains of subjects diagnosed with psychiatric disease, such as schizophrenia, depression or substance abuse.
Our neurogenomic approaches cover many aspects that relate to the cell nucleus, including gene expression, chromatin regulation etc. We do this in post-mortem brains (human tissue collected after death) as well as in mouse models of psychiatric disorders.
What is the current status of the field of epigenetics in regard to the understanding of 3D organization of the genome and why is this a key area to study?
Epigenetics is a very broad topic that is rapidly developing and in the areas of neurology and psychiatry research is generally following the main developments of the field. One of the more recent study subjects is the 3D ‘spatial organization’ of the genome inside each and every nucleus of our brain cells, which is challenging old views.
Imagine chromatin fibers inside the nucleus of differentiated cells (including brain cells and neurons) almost like a bunch spaghetti stuffed into a bowl. Each of these pasta noodles would be a chromosome with many millions of base pairs on it. Is such chromosome just stuffed randomly inside the cell nucleus without any clear organization? If you step back from your spaghetti dish and for one second think about each individual spaghetti as a chromosome, even the ones that have curved and coiled into themselves or are overlapping with other spaghettos, they do this, to a certain degree, in a non-random manner and therefore, there could be a lot of information in what we call the 3D or spatial conformation of the chromosomes inside the cell nucleus. That is what we are currently trying to study.
In order to truly understand the genome in full you have to look at smaller blocks of genomes that can be hundreds of thousands or sometimes millions of DNA base pairs long. Within individual sections, lots of genes and regulatory gene units are coregulated and, on top of that, each of these chromosomal domains is in potential interaction with similar types of domains located on other chromosomal loci.
For example, you may get a lot of information on genes or genomic loci that, by themselves, are not contributing to heritability risk for schizophrenia but are physically interacting with other loci that carry genetic risk. Therefore, mapping the ‘spatial genome’ in brain cells could help in our efforts to expand the genomic risk space important for psychiatric and neurological disorders and gain deeper understanding of genome biology and regulation of chromatin.
“One achievable goal for the field would be to generate, for the human brain, an as complete as possible atlas of functional non-coding DNA”
You recently worked on the PsychENCODE project, could you summarize the initial aims of the project?
PsychENCODE is a consortium sponsored by the National Institute of Mental Health and more recently also by the National Institute of Drug Abuse (both MD, USA). The consortium was formed with the main mission to improve our understanding of the role of the non-coding part of the genome for brain function and behavior, including psychiatric disease; that is all of the DNA not directly involved in encoding protein sequence and, until not too long ago, had been thought to be junk DNA. This includes 98.5% of the total genome.
What my research group did for PsychENCODE was to generate, for many hundreds of brains, genome-wide maps of histone acetylation and methylation landscapes, in order to inform about the activity status of the chromatin at any given gene. Simply put, actively expressed genes are defined by higher levels of acetylated histones in the surrounding chromatin.
Other PsychENCODE investigators have mapped DNA methylation, or gene expression levels, and various other molecular entities associated with genome function, and the biocomputational scientists in the consortium have conducted wonderful integrative analyses with datasets from nearly 2000 brains, thereby producing novel and disease-relevant gene regulatory networks, and even using brain genomic information to better define a complex psychiatric disease such as schizophrenia.
-
From Sanger sequencing to genome databases and beyond
-
Epigenetics and metabolism: what do we know?
-
The epigenetics of stress
The first series of the study was published at the beginning of this year, what are you working on now? What’s the next stage for the study?
On my ‘to-do’ list for PsychENCODE are brain cell types that are highly important for human behavior and function, but that remain, for various reasons, under-explored so far. A good example would be the dopaminergic neurons residing in the human midbrain. These often come in limited amounts in the postmortem brain tissue, so they are often underrepresented in genomic research. Dopaminergic neurons are important in everything from sleep to motivation to locomotion to cognition and they are involved in many neurological and psychiatric diseases.
What do you hope the end result of the study will be? Where do you hope to see this research in 5–10 years?
One achievable goal for the field would be to generate, for the human brain, an as complete as possible atlas of functional non-coding DNA, of the chromatin markings and of the various epigenetic markings (histone modifications, DNA methylation etc.), in as many different cell types and brain regions across as many different stages of development and aging. I think that will be an important task, as the wide range of neurological, neurodegenerative and psychiatric diseases each affect different brain cell types, yet a lot of heritability risk for human brain disorder is affecting non-coding DNA sequence.
Understanding genome organization and function in gene or chromosomal locus-specific manner and getting all of this data to relate back to the genetic risk architecture of specific patients may improve, in the hopefully not-so-distant future, current treatment options for the affected individuals.